Highly efficient transfer hydrogenation of carbonyl compounds over supported nickel and palladium nanoparticles
In this work, metal nanoparticles were successfully synthesized through the reduction of metal salts using reducing
agents such as: ethylene glycol (EG) and sodium borohydride (NaBH4). These metal nanoparticles were impregnated
into the supports M-X (M = Ni, Pd; X = Bent, C, Zeolite, and Al2O3) in high yield. The physio-chemical properties of
these catalysts were characterized by various techniques such as UV-Vis spectroscopy, powder X-ray diffraction
(PXRD), Transmission electron microscopy (TEM) and the specific surface area of M-X was evaluated by N2
adsorption isotherm analysis at 77 K. All results corroborated the loading process. Literally, TEM images indicated that
the palladium and nickel nanoparticles size are 6 and 13 nm, respectively. The efficiency of these catalysts was
performed on the transfer hydrogenation of various carbonyl substrates in the presence of potassium hydroxide at
atmosphere pressure. The results showed that both nickel and palladium supported X catalysts exhibited high activities
over 99 % within 60 min in the presence of potassium hydroxide.
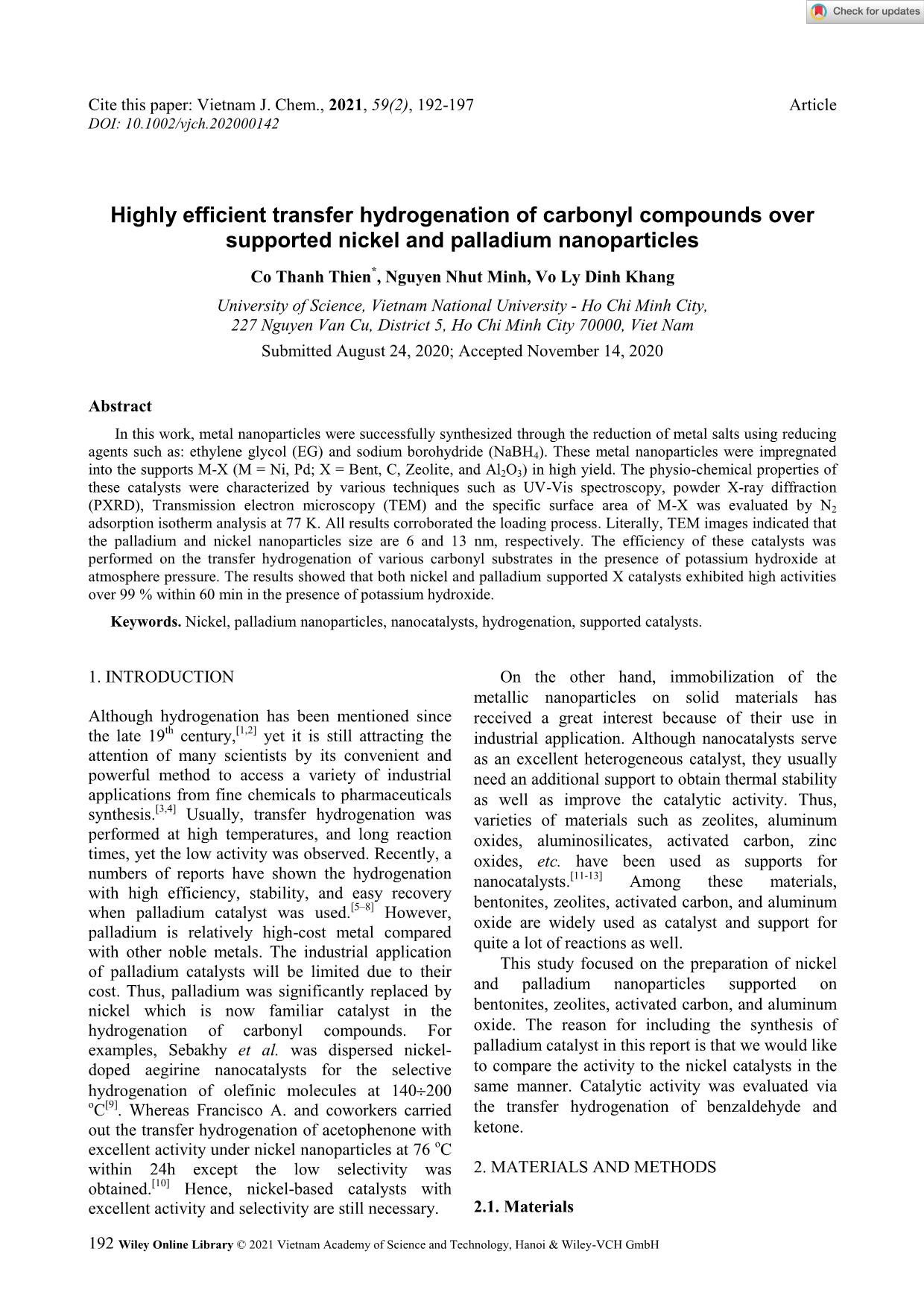
Trang 1
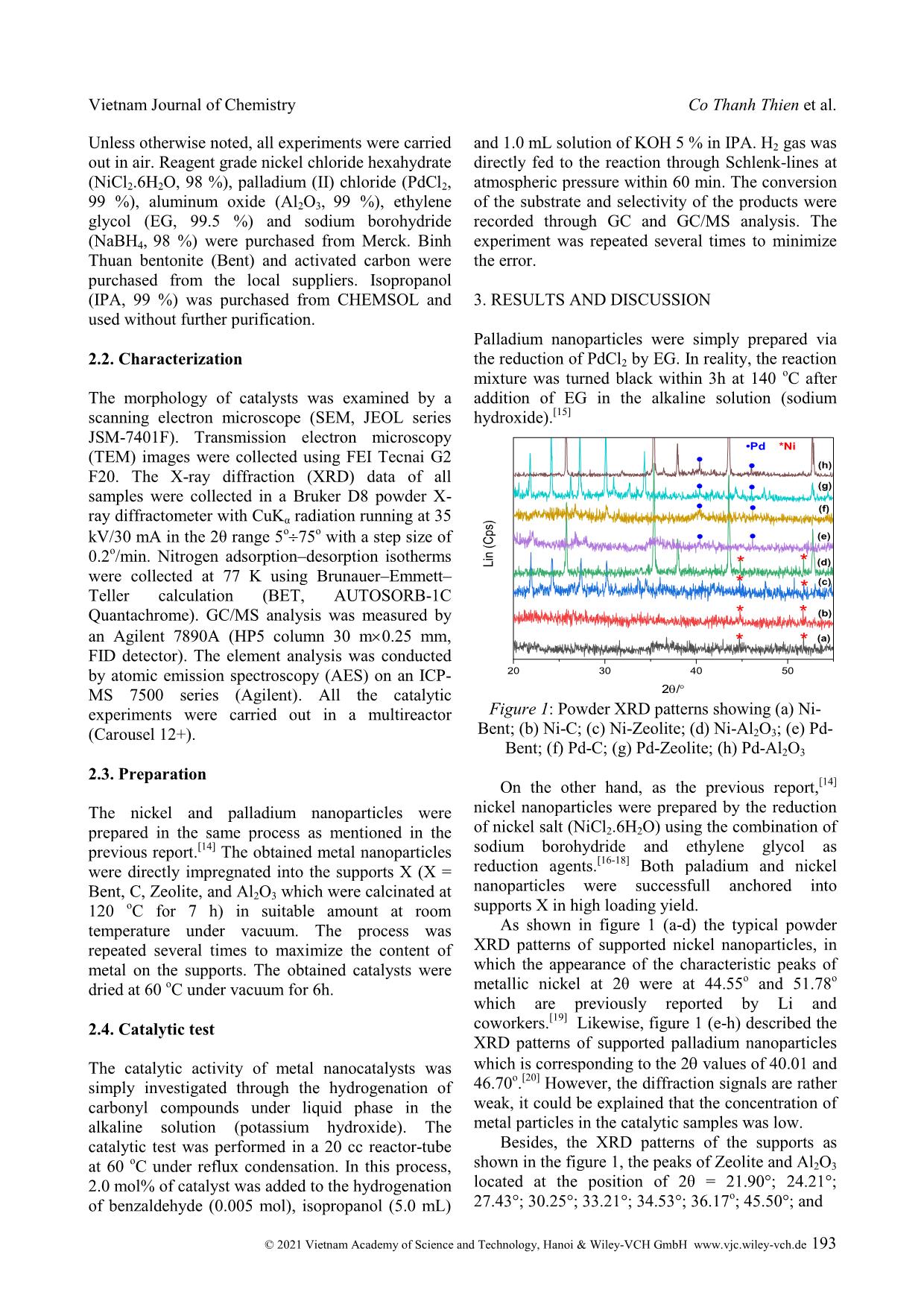
Trang 2
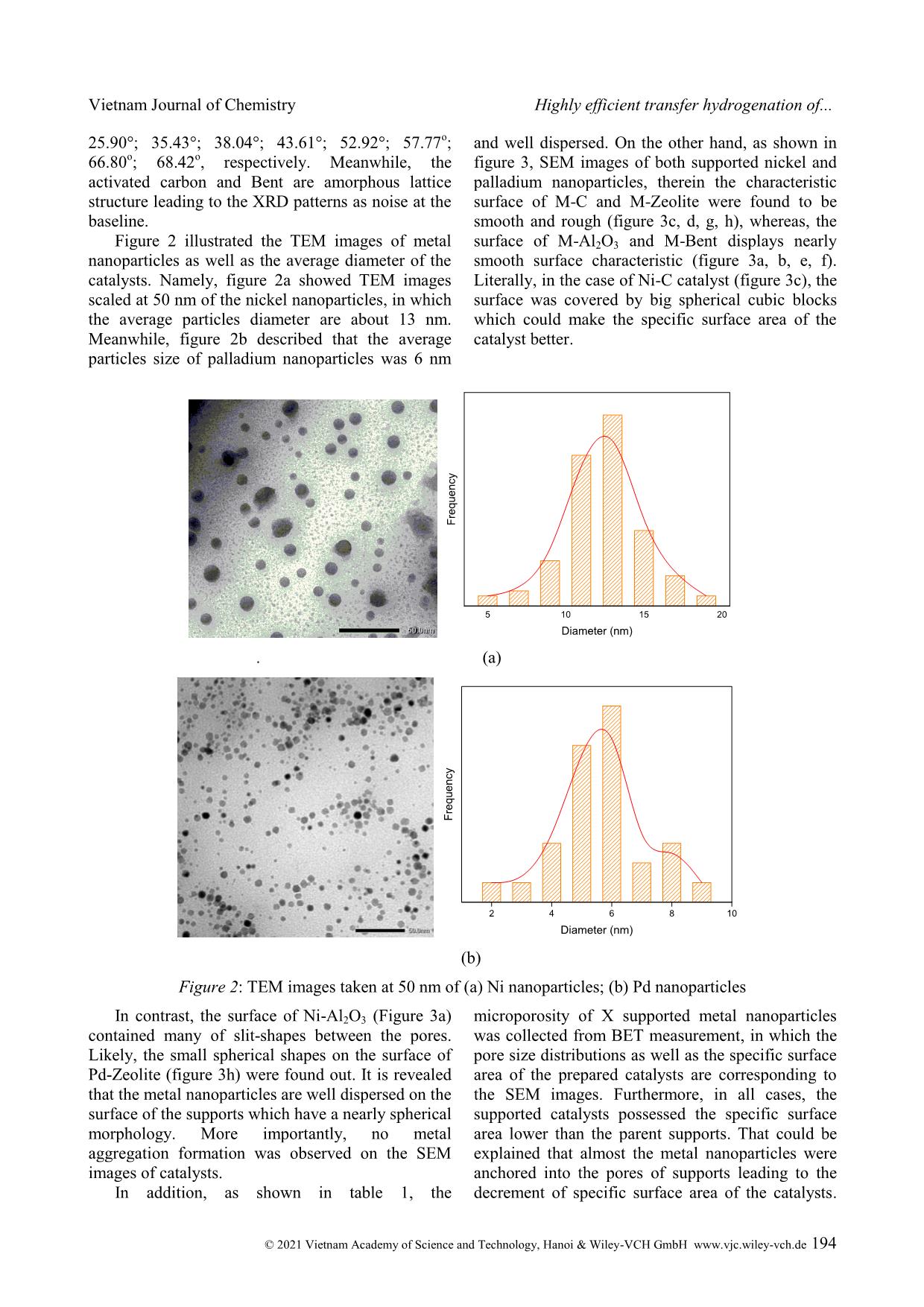
Trang 3
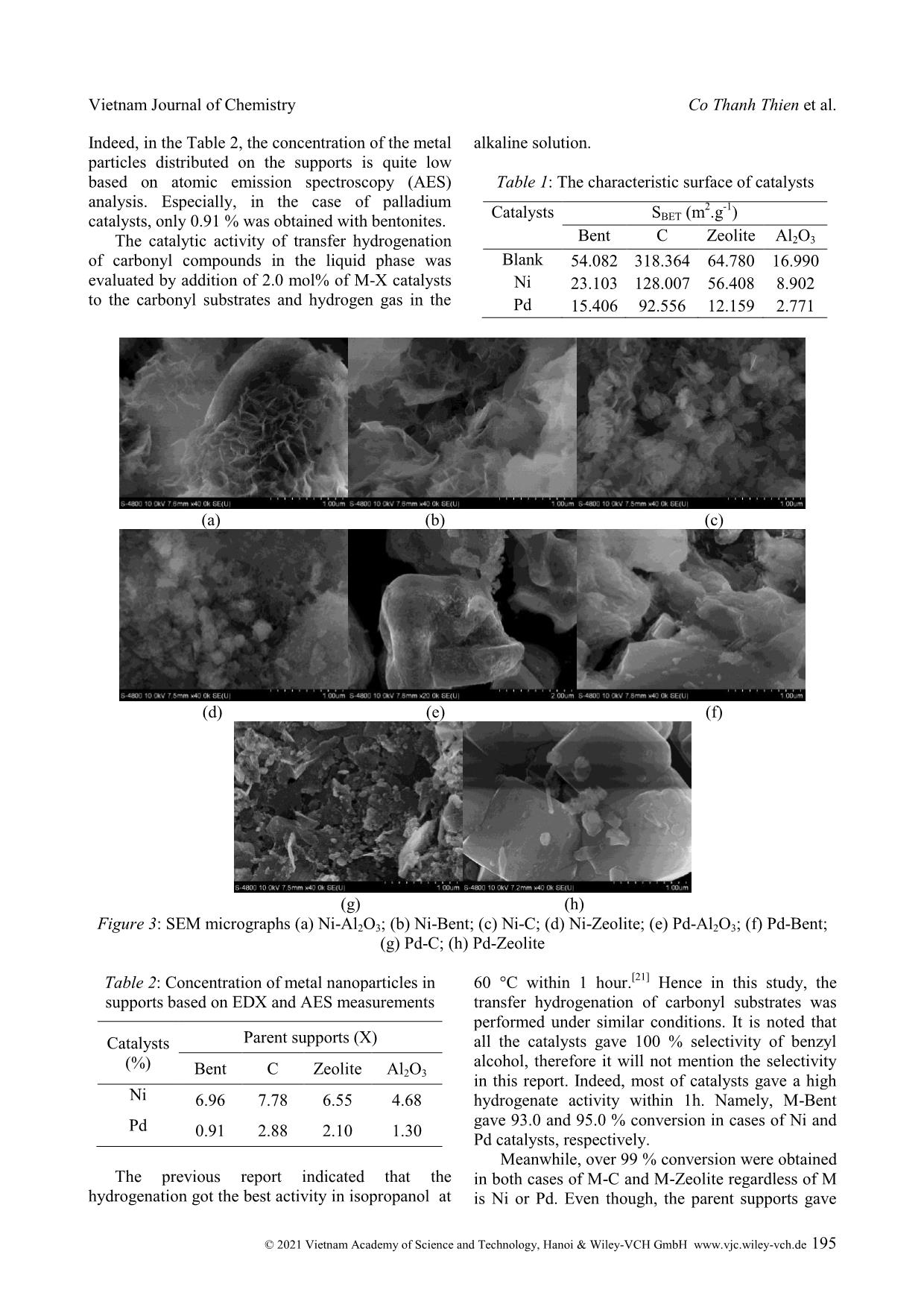
Trang 4
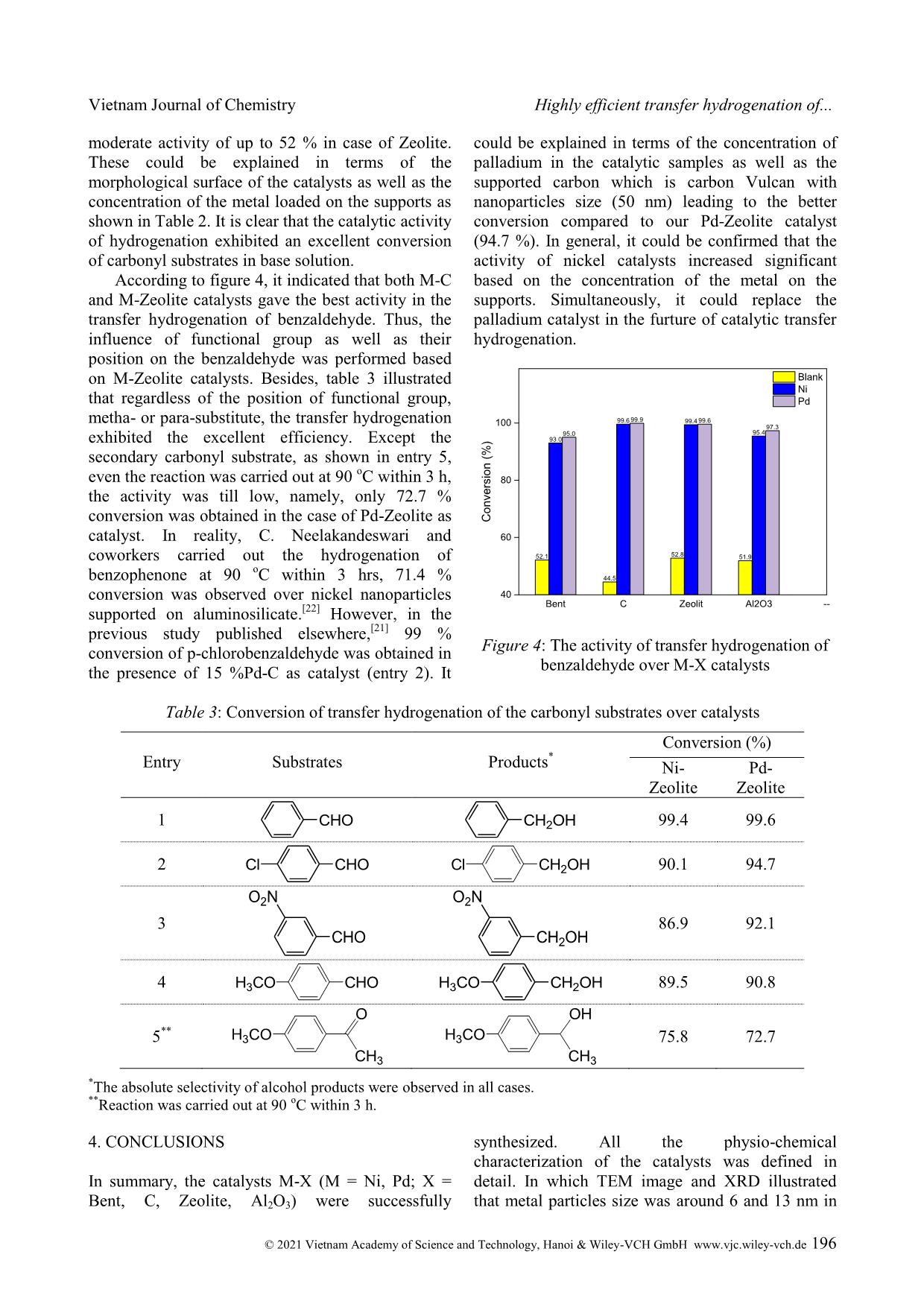
Trang 5
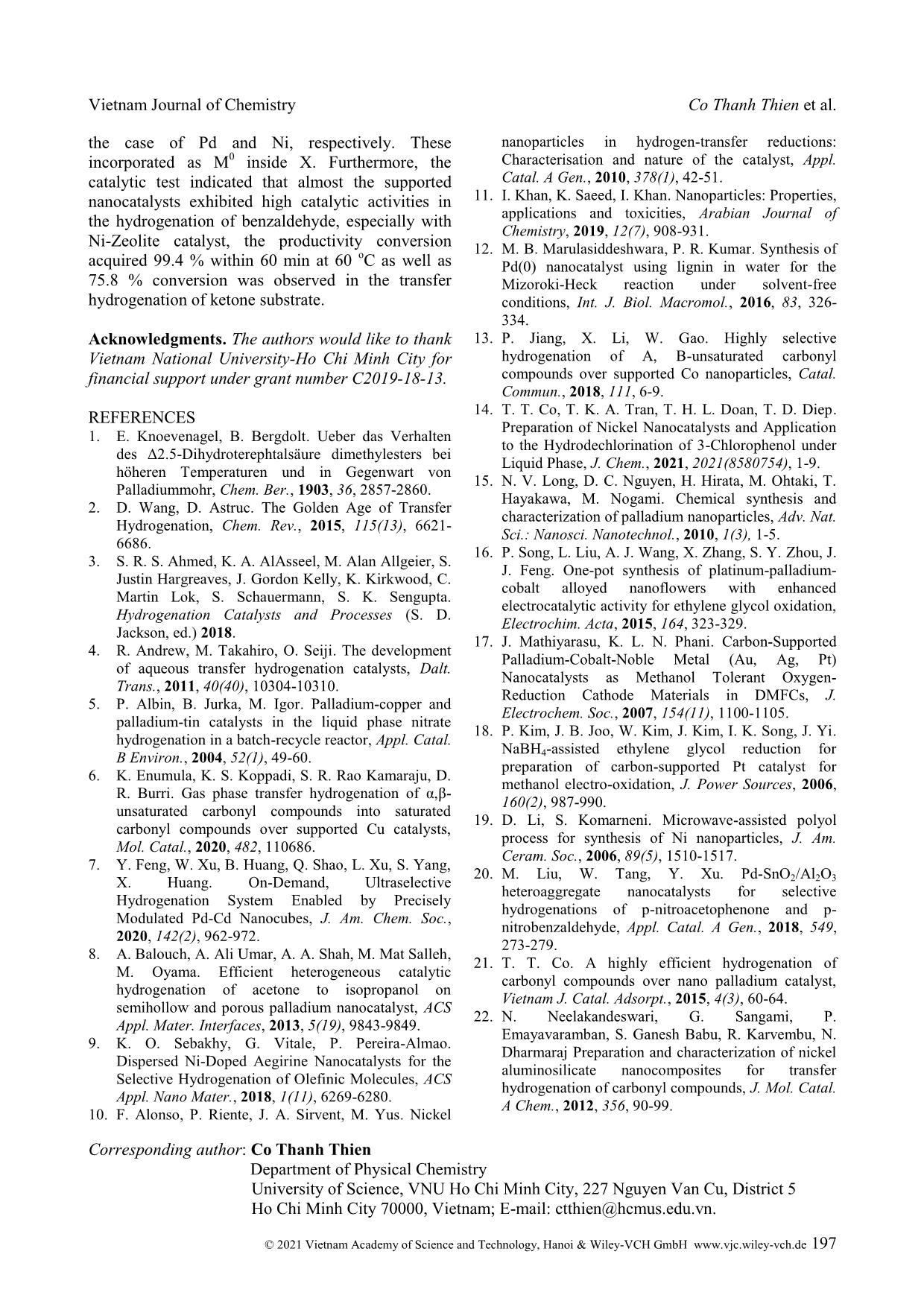
Trang 6
Tóm tắt nội dung tài liệu: Highly efficient transfer hydrogenation of carbonyl compounds over supported nickel and palladium nanoparticles
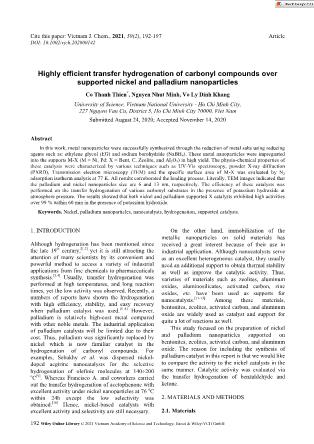
Cite this paper: Vietnam J. Chem., 2021, 59(2), 192-197 Article DOI: 10.1002/vjch.202000142 192 Wiley Online Library © 2021 Vietnam Academy of Science and Technology, Hanoi & Wiley-VCH GmbH Highly efficient transfer hydrogenation of carbonyl compounds over supported nickel and palladium nanoparticles Co Thanh Thien * , Nguyen Nhut Minh, Vo Ly Dinh Khang University of Science, Vietnam National University - Ho Chi Minh City, 227 Nguyen Van Cu, District 5, Ho Chi Minh City 70000, Viet Nam Submitted August 24, 2020; Accepted November 14, 2020 Abstract In this work, metal nanoparticles were successfully synthesized through the reduction of metal salts using reducing agents such as: ethylene glycol (EG) and sodium borohydride (NaBH4). These metal nanoparticles were impregnated into the supports M-X (M = Ni, Pd; X = Bent, C, Zeolite, and Al2O3) in high yield. The physio-chemical properties of these catalysts were characterized by various techniques such as UV-Vis spectroscopy, powder X-ray diffraction (PXRD), Transmission electron microscopy (TEM) and the specific surface area of M-X was evaluated by N2 adsorption isotherm analysis at 77 K. All results corroborated the loading process. Literally, TEM images indicated that the palladium and nickel nanoparticles size are 6 and 13 nm, respectively. The efficiency of these catalysts was performed on the transfer hydrogenation of various carbonyl substrates in the presence of potassium hydroxide at atmosphere pressure. The results showed that both nickel and palladium supported X catalysts exhibited high activities over 99 % within 60 min in the presence of potassium hydroxide. Keywords. Nickel, palladium nanoparticles, nanocatalysts, hydrogenation, supported catalysts. 1. INTRODUCTION Although hydrogenation has been mentioned since the late 19 th century, [1,2] yet it is still attracting the attention of many scientists by its convenient and powerful method to access a variety of industrial applications from fine chemicals to pharmaceuticals synthesis. [3,4] Usually, transfer hydrogenation was performed at high temperatures, and long reaction times, yet the low activity was observed. Recently, a numbers of reports have shown the hydrogenation with high efficiency, stability, and easy recovery when palladium catalyst was used. [5–8] However, palladium is relatively high-cost metal compared with other noble metals. The industrial application of palladium catalysts will be limited due to their cost. Thus, palladium was significantly replaced by nickel which is now familiar catalyst in the hydrogenation of carbonyl compounds. For examples, Sebakhy et al. was dispersed nickel- doped aegirine nanocatalysts for the selective hydrogenation of olefinic molecules at 140200 o C [9] . Whereas Francisco A. and coworkers carried out the transfer hydrogenation of acetophenone with excellent activity under nickel nanoparticles at 76 o C within 24h except the low selectivity was obtained. [10] Hence, nickel-based catalysts with excellent activity and selectivity are still necessary. On the other hand, immobilization of the metallic nanoparticles on solid materials has received a great interest because of their use in industrial application. Although nanocatalysts serve as an excellent heterogeneous catalyst, they usually need an additional support to obtain thermal stability as well as improve the catalytic activity. Thus, varieties of materials such as zeolites, aluminum oxides, aluminosilicates, activated carbon, zinc oxides, etc. have been used as supports for nanocatalysts. [11-13] Among these materials, bentonites, zeolites, activated carbon, and aluminum oxide are widely used as catalyst and support for quite a lot of reactions as well. This study focused on the preparation of nickel and palladium nanoparticles supported on bentonites, zeolites, activated carbon, and aluminum oxide. The reason for including the synthesis of palladium catalyst in this report is that we would like to compare the activity to the nickel catalysts in the same manner. Catalytic activity was evaluated via the transfer hydrogenation of benzaldehyde and ketone. 2. MATERIALS AND METHODS 2.1. Materials Vietnam Journal of Chemistry Co Thanh Thien et al. © 2021 Vietnam Academy of Science and Technology, Hanoi & Wiley-VCH GmbH www.vjc.wiley-vch.de 193 Unless otherwise noted, all experiments were carried out in air. Reagent grade nickel chloride hexahydrate (NiCl2.6H2O, 98 %), palladium (II) chloride (PdCl2, 99 %), aluminum oxide (Al2O3, 99 %), ethylene glycol (EG, 99.5 %) and sodium borohydride (NaBH4, 98 %) were purchased from Merck. Binh Thuan bentonite (Bent) and activated carbon were purchased from the local suppliers. Isopropanol (IPA, 99 %) was purchased from CHEMSOL and used without further purification. 2.2. Characterization The morphology of catalysts was examined by a scanning electron microscope (SEM, JEOL series JSM-7401F). Transmission electron microscopy (TEM) images were collected using FEI Tecnai G2 F20. The X-ray diffraction (XRD) data of all samples were collected in a Bruker D8 powder X- ray diffractometer with CuKα radiation running at 35 kV/30 mA in the 2θ range 5o75o with a step size of 0.2 o /min. Nitrogen adsorption–desorption isotherms were collected at 77 K using Brunauer–Emmett– Teller calculation (BET, AUTOSORB-1C Quantachrome). GC/MS analysis was measured by an Agilent 7890A (HP5 column 30 m 0.25 mm, FID detector). The element analysis was conducted by atomic emission spectroscopy (AES) on an ICP- MS 7500 series (Agilent). All the catalytic experiments were carried out in a multireactor (Carousel 12+). 2.3. Preparation The nickel and palladium nanoparticles were prepared in the same process as mentioned in the previous repor ... Both paladium and nickel nanoparticles were successfull anchored into supports X in high loading yield. As shown in figure 1 (a-d) the typical powder XRD patterns of supported nickel nanoparticles, in which the appearance of the characteristic peaks of metallic nickel at 2θ were at 44.55o and 51.78o which are previously reported by Li and coworkers. [19] Likewise, figure 1 (e-h) described the XRD patterns of supported palladium nanoparticles which is corresponding to the 2q values of 40.01 and 46.70 o . [20] However, the diffraction signals are rather weak, it could be explained that the concentration of metal particles in the catalytic samples was low. Besides, the XRD patterns of the supports as shown in the figure 1, the peaks of Zeolite and Al2O3 located at the position of 2θ = 21.90°; 24.21°; 27.43°; 30.25°; 33.21°; 34.53°; 36.17 o ; 45.50°; and Vietnam Journal of Chemistry Highly efficient transfer hydrogenation of... © 2021 Vietnam Academy of Science and Technology, Hanoi & Wiley-VCH GmbH www.vjc.wiley-vch.de 194 25.90°; 35.43°; 38.04°; 43.61°; 52.92°; 57.77 o ; 66.80 o ; 68.42 o , respectively. Meanwhile, the activated carbon and Bent are amorphous lattice structure leading to the XRD patterns as noise at the baseline. Figure 2 illustrated the TEM images of metal nanoparticles as well as the average diameter of the catalysts. Namely, figure 2a showed TEM images scaled at 50 nm of the nickel nanoparticles, in which the average particles diameter are about 13 nm. Meanwhile, figure 2b described that the average particles size of palladium nanoparticles was 6 nm and well dispersed. On the other hand, as shown in figure 3, SEM images of both supported nickel and palladium nanoparticles, therein the characteristic surface of M-C and M-Zeolite were found to be smooth and rough (figure 3c, d, g, h), whereas, the surface of M-Al2O3 and M-Bent displays nearly smooth surface characteristic (figure 3a, b, e, f). Literally, in the case of Ni-C catalyst (figure 3c), the surface was covered by big spherical cubic blocks which could make the specific surface area of the catalyst better. 5 10 15 20 F re q u e n c y Diameter (nm) . (a) 2 4 6 8 10 F re q u e n c y Diameter (nm) (b) Figure 2: TEM images taken at 50 nm of (a) Ni nanoparticles; (b) Pd nanoparticles In contrast, the surface of Ni-Al2O3 (Figure 3a) contained many of slit-shapes between the pores. Likely, the small spherical shapes on the surface of Pd-Zeolite (figure 3h) were found out. It is revealed that the metal nanoparticles are well dispersed on the surface of the supports which have a nearly spherical morphology. More importantly, no metal aggregation formation was observed on the SEM images of catalysts. In addition, as shown in table 1, the microporosity of X supported metal nanoparticles was collected from BET measurement, in which the pore size distributions as well as the specific surface area of the prepared catalysts are corresponding to the SEM images. Furthermore, in all cases, the supported catalysts possessed the specific surface area lower than the parent supports. That could be explained that almost the metal nanoparticles were anchored into the pores of supports leading to the decrement of specific surface area of the catalysts. Vietnam Journal of Chemistry Co Thanh Thien et al. © 2021 Vietnam Academy of Science and Technology, Hanoi & Wiley-VCH GmbH www.vjc.wiley-vch.de 195 Indeed, in the Table 2, the concentration of the metal particles distributed on the supports is quite low based on atomic emission spectroscopy (AES) analysis. Especially, in the case of palladium catalysts, only 0.91 % was obtained with bentonites. The catalytic activity of transfer hydrogenation of carbonyl compounds in the liquid phase was evaluated by addition of 2.0 mol% of M-X catalysts to the carbonyl substrates and hydrogen gas in the alkaline solution. Table 1: The characteristic surface of catalysts Catalysts SBET (m 2 .g -1 ) Bent C Zeolite Al2O3 Blank 54.082 318.364 64.780 16.990 Ni 23.103 128.007 56.408 8.902 Pd 15.406 92.556 12.159 2.771 (a) (b) (c) (d) (e) (f) (g) (h) Figure 3: SEM micrographs (a) Ni-Al2O3; (b) Ni-Bent; (c) Ni-C; (d) Ni-Zeolite; (e) Pd-Al2O3; (f) Pd-Bent; (g) Pd-C; (h) Pd-Zeolite Table 2: Concentration of metal nanoparticles in supports based on EDX and AES measurements Catalysts (%) Parent supports (X) Bent C Zeolite Al2O3 Ni 6.96 7.78 6.55 4.68 Pd 0.91 2.88 2.10 1.30 The previous report indicated that the hydrogenation got the best activity in isopropanol at 60 °C within 1 hour. [21] Hence in this study, the transfer hydrogenation of carbonyl substrates was performed under similar conditions. It is noted that all the catalysts gave 100 % selectivity of benzyl alcohol, therefore it will not mention the selectivity in this report. Indeed, most of catalysts gave a high hydrogenate activity within 1h. Namely, M-Bent gave 93.0 and 95.0 % conversion in cases of Ni and Pd catalysts, respectively. Meanwhile, over 99 % conversion were obtained in both cases of M-C and M-Zeolite regardless of M is Ni or Pd. Even though, the parent supports gave Vietnam Journal of Chemistry Highly efficient transfer hydrogenation of... © 2021 Vietnam Academy of Science and Technology, Hanoi & Wiley-VCH GmbH www.vjc.wiley-vch.de 196 moderate activity of up to 52 % in case of Zeolite. These could be explained in terms of the morphological surface of the catalysts as well as the concentration of the metal loaded on the supports as shown in Table 2. It is clear that the catalytic activity of hydrogenation exhibited an excellent conversion of carbonyl substrates in base solution. According to figure 4, it indicated that both M-C and M-Zeolite catalysts gave the best activity in the transfer hydrogenation of benzaldehyde. Thus, the influence of functional group as well as their position on the benzaldehyde was performed based on M-Zeolite catalysts. Besides, table 3 illustrated that regardless of the position of functional group, metha- or para-substitute, the transfer hydrogenation exhibited the excellent efficiency. Except the secondary carbonyl substrate, as shown in entry 5, even the reaction was carried out at 90 o C within 3 h, the activity was till low, namely, only 72.7 % conversion was obtained in the case of Pd-Zeolite as catalyst. In reality, C. Neelakandeswari and coworkers carried out the hydrogenation of benzophenone at 90 o C within 3 hrs, 71.4 % conversion was observed over nickel nanoparticles supported on aluminosilicate. [22] However, in the previous study published elsewhere, [21] 99 % conversion of p-chlorobenzaldehyde was obtained in the presence of 15 %Pd-C as catalyst (entry 2). It could be explained in terms of the concentration of palladium in the catalytic samples as well as the supported carbon which is carbon Vulcan with nanoparticles size (50 nm) leading to the better conversion compared to our Pd-Zeolite catalyst (94.7 %). In general, it could be confirmed that the activity of nickel catalysts increased significant based on the concentration of the metal on the supports. Simultaneously, it could replace the palladium catalyst in the furture of catalytic transfer hydrogenation. 52.1 44.5 52.8 51.9 93.0 99.6 99.4 95.495.0 99.9 99.6 97.3 Bent C Zeolit Al2O3 -- 40 60 80 100 C o n v e rs io n ( % ) Blank Ni Pd Figure 4: The activity of transfer hydrogenation of benzaldehyde over M-X catalysts Table 3: Conversion of transfer hydrogenation of the carbonyl substrates over catalysts Entry Substrates Products * Conversion (%) Ni- Zeolite Pd- Zeolite 1 CHO CH2OH 99.4 99.6 2 CHOCl CH2OHCl 90.1 94.7 3 CHO O2N CH2OH O2N 86.9 92.1 4 CHOH3CO CH2OHH3CO 89.5 90.8 5 ** H3CO O CH3 H3CO OH CH3 75.8 72.7 * The absolute selectivity of alcohol products were observed in all cases. ** Reaction was carried out at 90 o C within 3 h. 4. CONCLUSIONS In summary, the catalysts M-X (M = Ni, Pd; X = Bent, C, Zeolite, Al2O3) were successfully synthesized. All the physio-chemical characterization of the catalysts was defined in detail. In which TEM image and XRD illustrated that metal particles size was around 6 and 13 nm in Vietnam Journal of Chemistry Co Thanh Thien et al. © 2021 Vietnam Academy of Science and Technology, Hanoi & Wiley-VCH GmbH www.vjc.wiley-vch.de 197 the case of Pd and Ni, respectively. These incorporated as M 0 inside X. Furthermore, the catalytic test indicated that almost the supported nanocatalysts exhibited high catalytic activities in the hydrogenation of benzaldehyde, especially with Ni-Zeolite catalyst, the productivity conversion acquired 99.4 % within 60 min at 60 o C as well as 75.8 % conversion was observed in the transfer hydrogenation of ketone substrate. Acknowledgments. The authors would like to thank Vietnam National University-Ho Chi Minh City for financial support under grant number C2019-18-13. REFERENCES 1. E. Knoevenagel, B. Bergdolt. Ueber das Verhalten des Γ2.5-Dihydroterephtalsäure dimethylesters bei höheren Temperaturen und in Gegenwart von Palladiummohr, Chem. Ber., 1903, 36, 2857-2860. 2. D. Wang, D. Astruc. The Golden Age of Transfer Hydrogenation, Chem. Rev., 2015, 115(13), 6621- 6686. 3. S. R. S. Ahmed, K. A. AlAsseel, M. Alan Allgeier, S. Justin Hargreaves, J. Gordon Kelly, K. Kirkwood, C. Martin Lok, S. Schauermann, S. K. Sengupta. Hydrogenation Catalysts and Processes (S. D. Jackson, ed.) 2018. 4. R. Andrew, M. Takahiro, O. Seiji. The development of aqueous transfer hydrogenation catalysts, Dalt. Trans., 2011, 40(40), 10304-10310. 5. P. Albin, B. Jurka, M. Igor. Palladium-copper and palladium-tin catalysts in the liquid phase nitrate hydrogenation in a batch-recycle reactor, Appl. Catal. B Environ., 2004, 52(1), 49-60. 6. K. Enumula, K. S. Koppadi, S. R. Rao Kamaraju, D. R. Burri. Gas phase transfer hydrogenation of α,β- unsaturated carbonyl compounds into saturated carbonyl compounds over supported Cu catalysts, Mol. Catal., 2020, 482, 110686. 7. Y. Feng, W. Xu, B. Huang, Q. Shao, L. Xu, S. Yang, X. Huang. On-Demand, Ultraselective Hydrogenation System Enabled by Precisely Modulated Pd-Cd Nanocubes, J. Am. Chem. Soc., 2020, 142(2), 962-972. 8. A. Balouch, A. Ali Umar, A. A. Shah, M. Mat Salleh, M. Oyama. Efficient heterogeneous catalytic hydrogenation of acetone to isopropanol on semihollow and porous palladium nanocatalyst, ACS Appl. Mater. Interfaces, 2013, 5(19), 9843-9849. 9. K. O. Sebakhy, G. Vitale, P. Pereira-Almao. Dispersed Ni-Doped Aegirine Nanocatalysts for the Selective Hydrogenation of Olefinic Molecules, ACS Appl. Nano Mater., 2018, 1(11), 6269-6280. 10. F. Alonso, P. Riente, J. A. Sirvent, M. Yus. Nickel nanoparticles in hydrogen-transfer reductions: Characterisation and nature of the catalyst, Appl. Catal. A Gen., 2010, 378(1), 42-51. 11. I. Khan, K. Saeed, I. Khan. Nanoparticles: Properties, applications and toxicities, Arabian Journal of Chemistry, 2019, 12(7), 908-931. 12. M. B. Marulasiddeshwara, P. R. Kumar. Synthesis of Pd(0) nanocatalyst using lignin in water for the Mizoroki-Heck reaction under solvent-free conditions, Int. J. Biol. Macromol., 2016, 83, 326- 334. 13. P. Jiang, X. Li, W. Gao. Highly selective hydrogenation of Α, Β-unsaturated carbonyl compounds over supported Co nanoparticles, Catal. Commun., 2018, 111, 6-9. 14. T. T. Co, T. K. A. Tran, T. H. L. Doan, T. D. Diep. Preparation of Nickel Nanocatalysts and Application to the Hydrodechlorination of 3-Chlorophenol under Liquid Phase, J. Chem., 2021, 2021(8580754), 1-9. 15. N. V. Long, D. C. Nguyen, H. Hirata, M. Ohtaki, T. Hayakawa, M. Nogami. Chemical synthesis and characterization of palladium nanoparticles, Adv. Nat. Sci.: Nanosci. Nanotechnol., 2010, 1(3), 1-5. 16. P. Song, L. Liu, A. J. Wang, X. Zhang, S. Y. Zhou, J. J. Feng. One-pot synthesis of platinum-palladium- cobalt alloyed nanoflowers with enhanced electrocatalytic activity for ethylene glycol oxidation, Electrochim. Acta, 2015, 164, 323-329. 17. J. Mathiyarasu, K. L. N. Phani. Carbon-Supported Palladium-Cobalt-Noble Metal (Au, Ag, Pt) Nanocatalysts as Methanol Tolerant Oxygen- Reduction Cathode Materials in DMFCs, J. Electrochem. Soc., 2007, 154(11), 1100-1105. 18. P. Kim, J. B. Joo, W. Kim, J. Kim, I. K. Song, J. Yi. NaBH4-assisted ethylene glycol reduction for preparation of carbon-supported Pt catalyst for methanol electro-oxidation, J. Power Sources, 2006, 160(2), 987-990. 19. D. Li, S. Komarneni. Microwave-assisted polyol process for synthesis of Ni nanoparticles, J. Am. Ceram. Soc., 2006, 89(5), 1510-1517. 20. M. Liu, W. Tang, Y. Xu. Pd-SnO2/Al2O3 heteroaggregate nanocatalysts for selective hydrogenations of p-nitroacetophenone and p- nitrobenzaldehyde, Appl. Catal. A Gen., 2018, 549, 273-279. 21. T. T. Co. A highly efficient hydrogenation of carbonyl compounds over nano palladium catalyst, Vietnam J. Catal. Adsorpt., 2015, 4(3), 60-64. 22. N. Neelakandeswari, G. Sangami, P. Emayavaramban, S. Ganesh Babu, R. Karvembu, N. Dharmaraj Preparation and characterization of nickel aluminosilicate nanocomposites for transfer hydrogenation of carbonyl compounds, J. Mol. Catal. A Chem., 2012, 356, 90-99. Corresponding author: Co Thanh Thien Department of Physical Chemistry University of Science, VNU Ho Chi Minh City, 227 Nguyen Van Cu, District 5 Ho Chi Minh City 70000, Vietnam; E-mail: ctthien@hcmus.edu.vn.
File đính kèm:
highly_efficient_transfer_hydrogenation_of_carbonyl_compound.pdf