Fabrication and lithium storage performances of a composite of tin disulfide and ordered mesoporous carbon
SnS2 has been investigated as anode material for lithium ion batteries. To obtain anode material having high
capacity and long-term lifespan, in the present work, a nanostructured composite comprising SnS2 nanosheets and
CMK-3 ordered mesoporous carbon has been designed. The CMK-3/SnS2 composite is fabricated via incipient wetness
impregnation, followed by chemical reduction and chemical conversion in an inert gas at high temperature. The
obtained composite exhibits boosted lithium storage behaviors involving high specific capacity, fast rate response and
stable cyclability. At a discharge-charge rate of 100 mA g-1, the CMK-3/SnS2 electrode delivers a specific capacity of
985.2 mA h g-1 with the utilization efficiency of SnS2 present in the composite of 90.5 %. Even, after 500 cycles testing
at higher discharge-charge rates of 0.5 and 1 A g-1, CMK-3/SnS2 still can maintain specific capacities of 556.2 and
402.9 mA h g-1, respectively, much higher than the theoretical specific capacity of commercialized graphite anode
material. This work demonstrates considerable application potential of the CMK-3/SnS2 anode in Li-ion batteries.
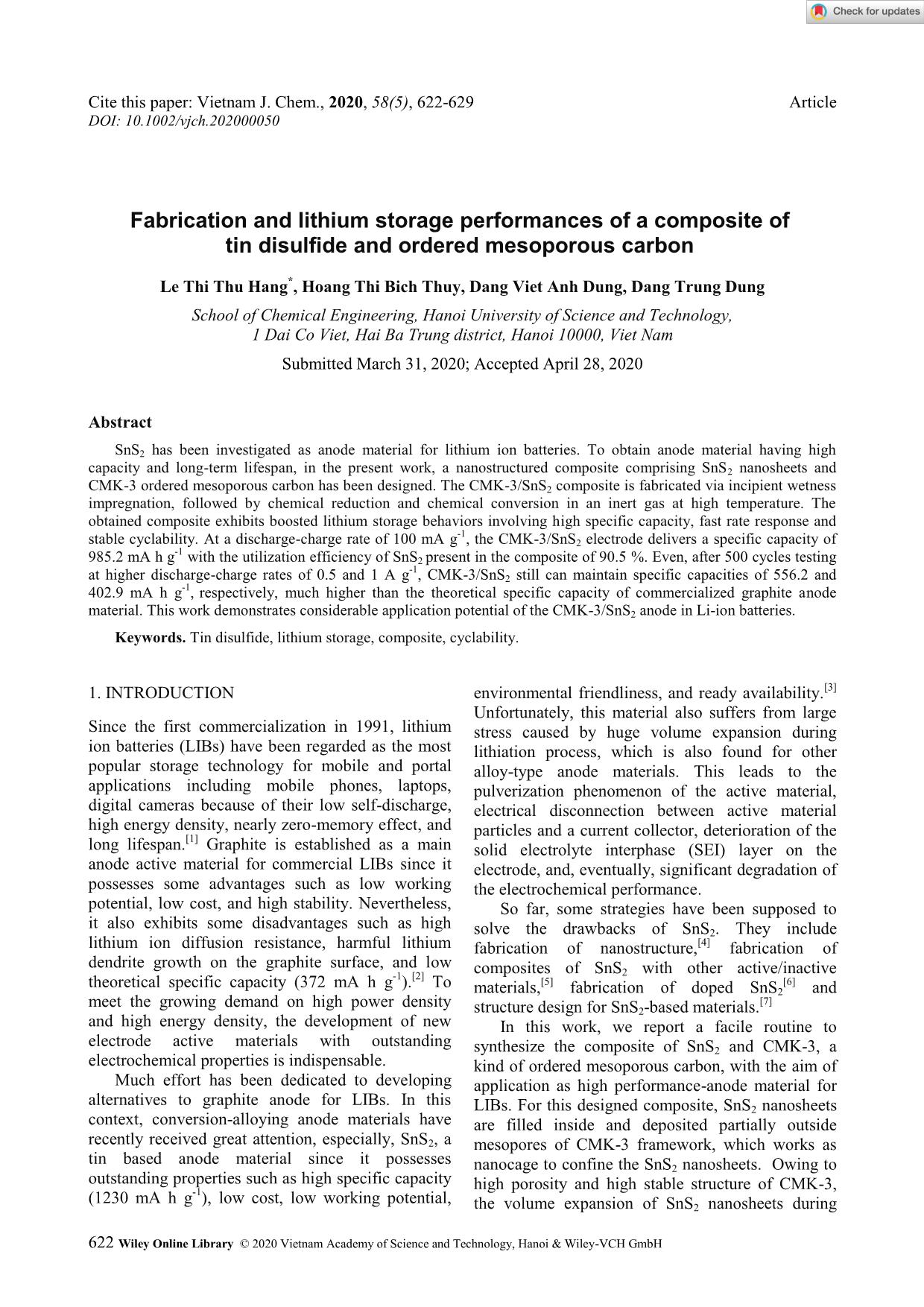
Trang 1
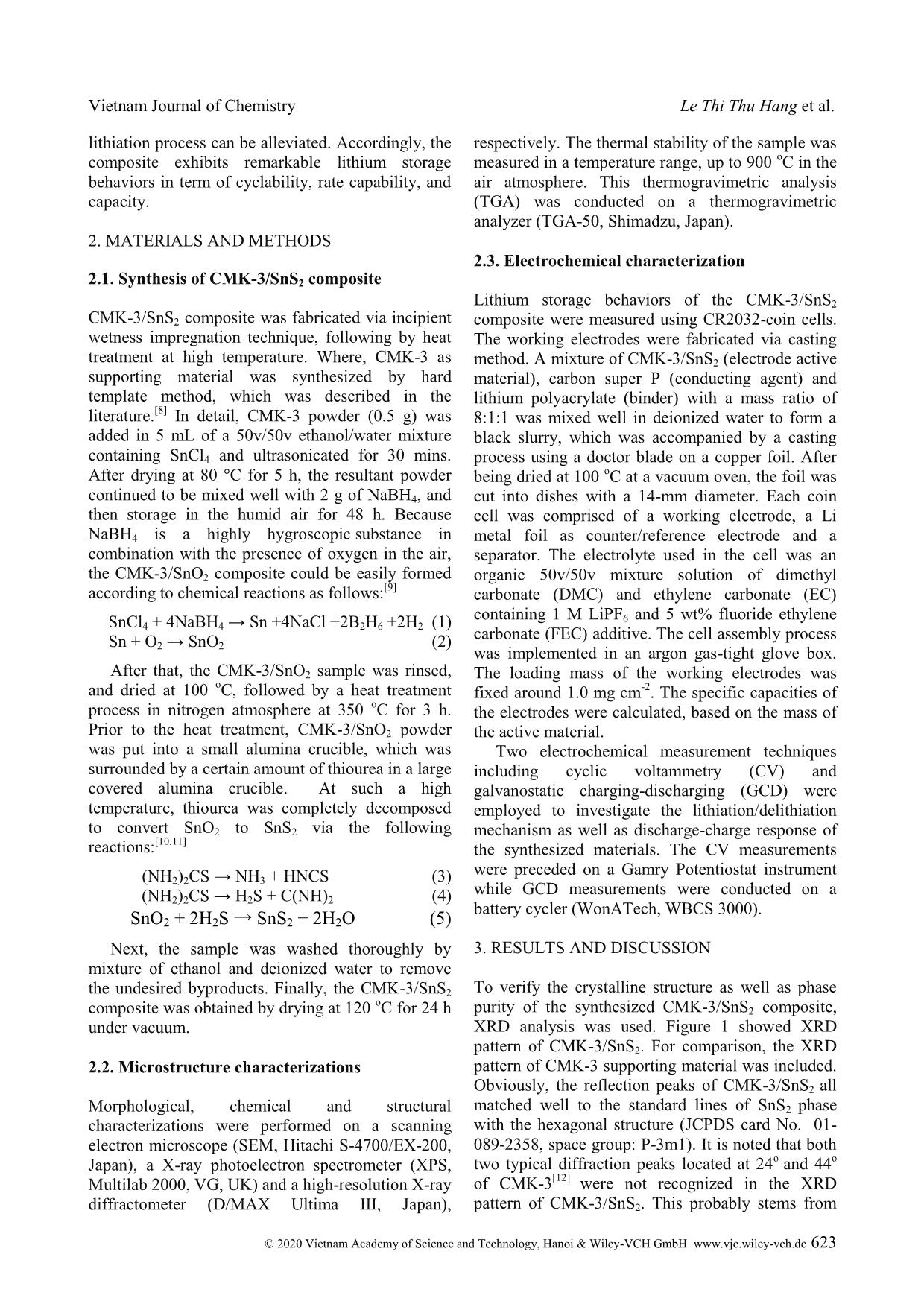
Trang 2
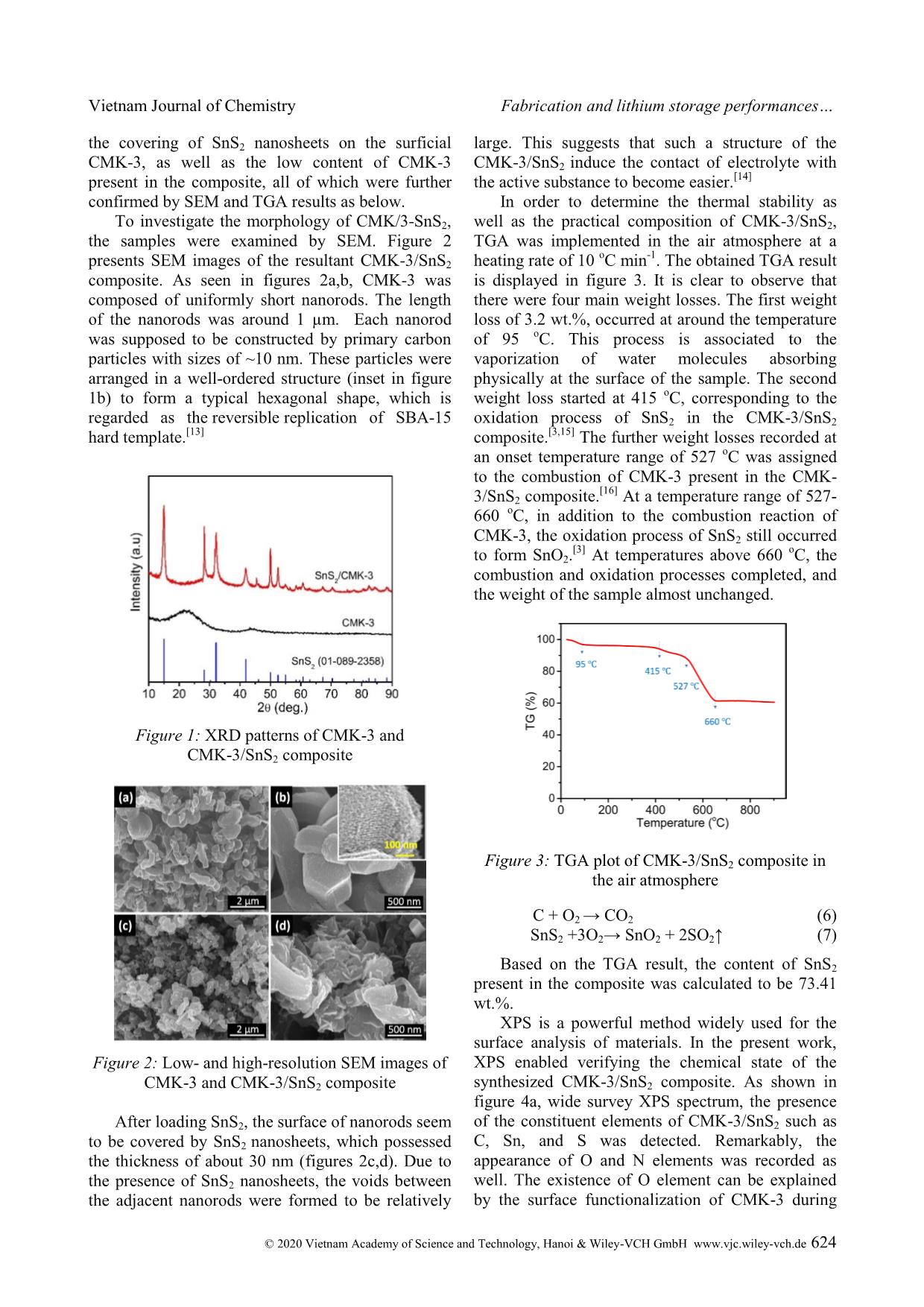
Trang 3
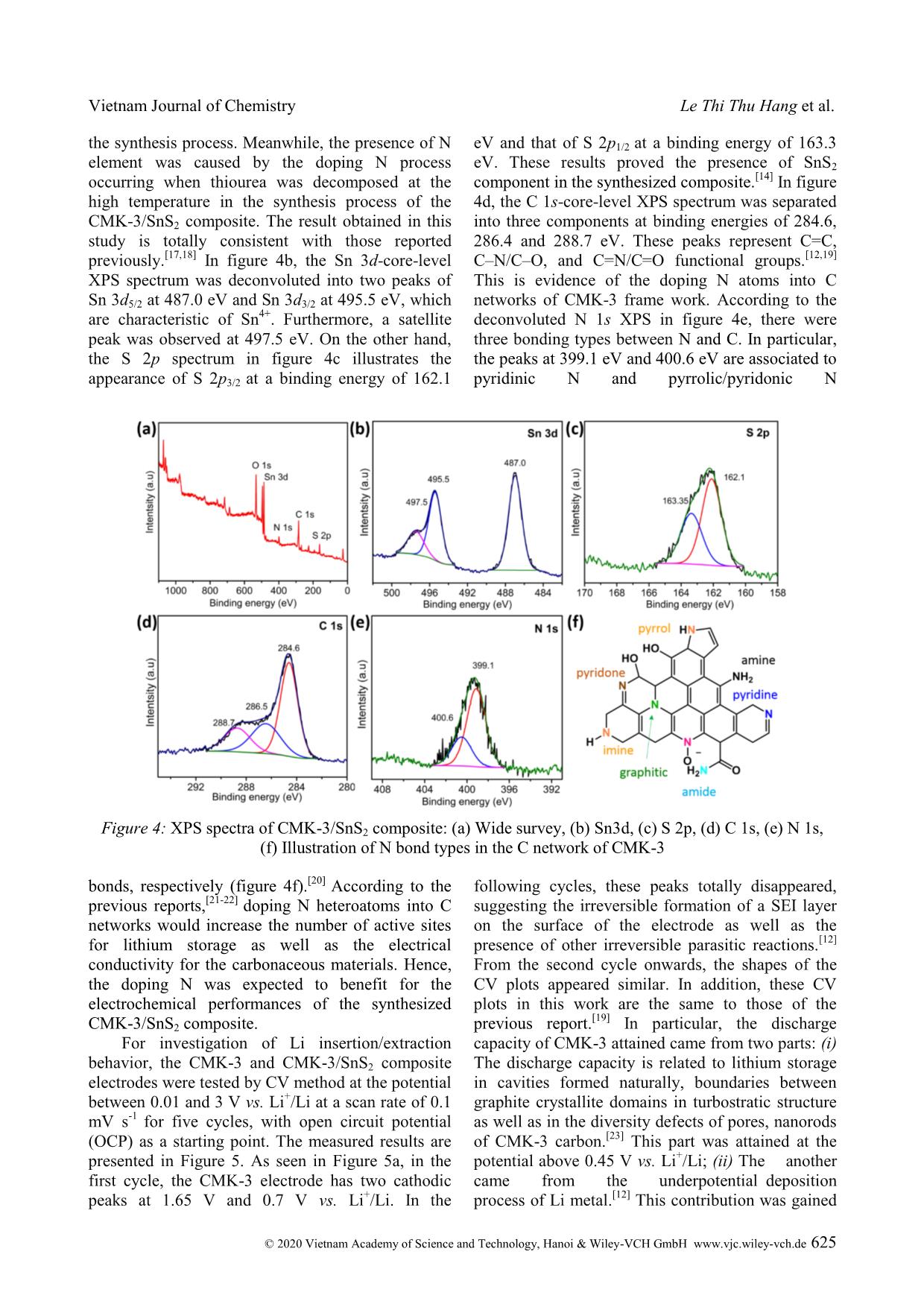
Trang 4
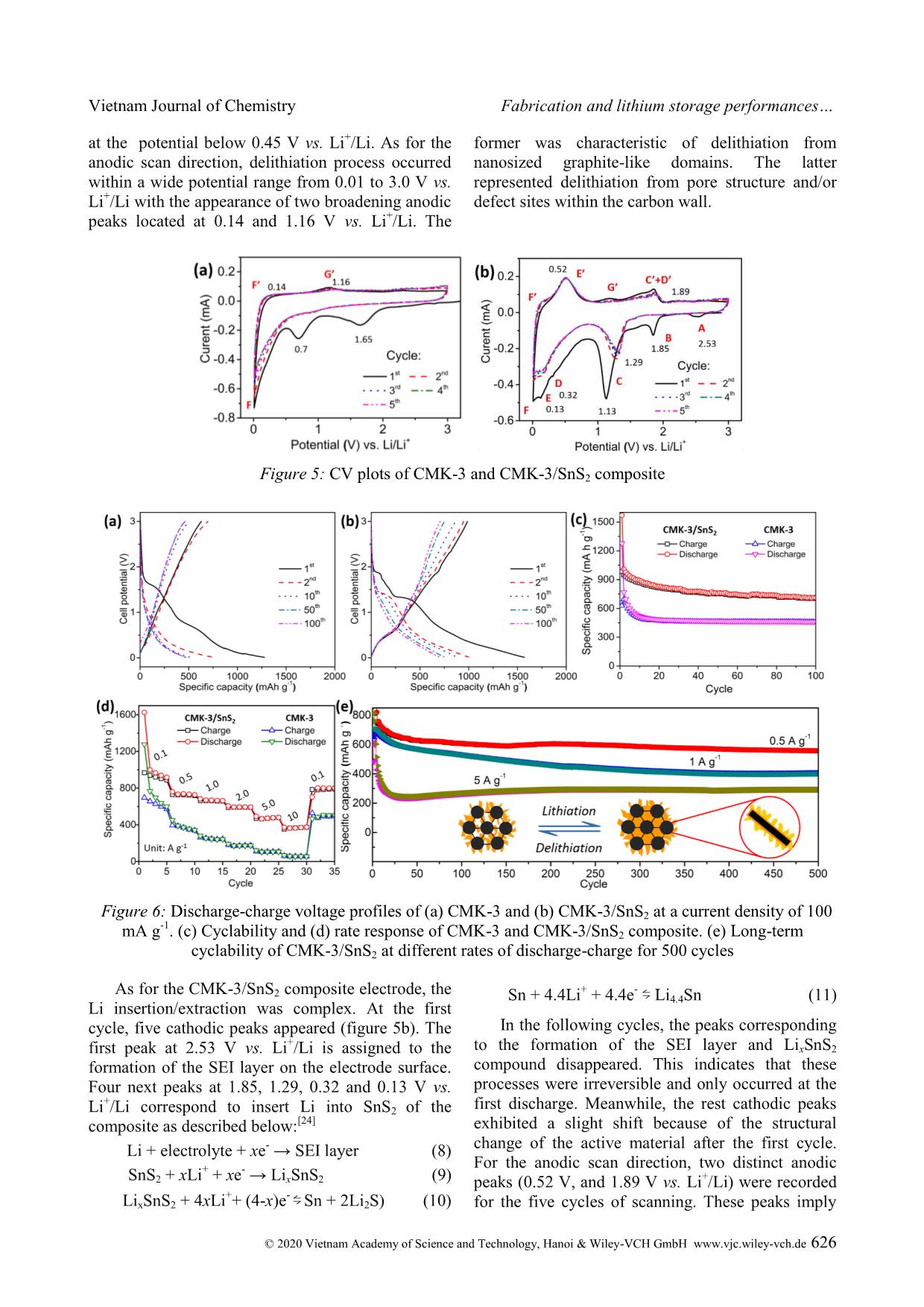
Trang 5
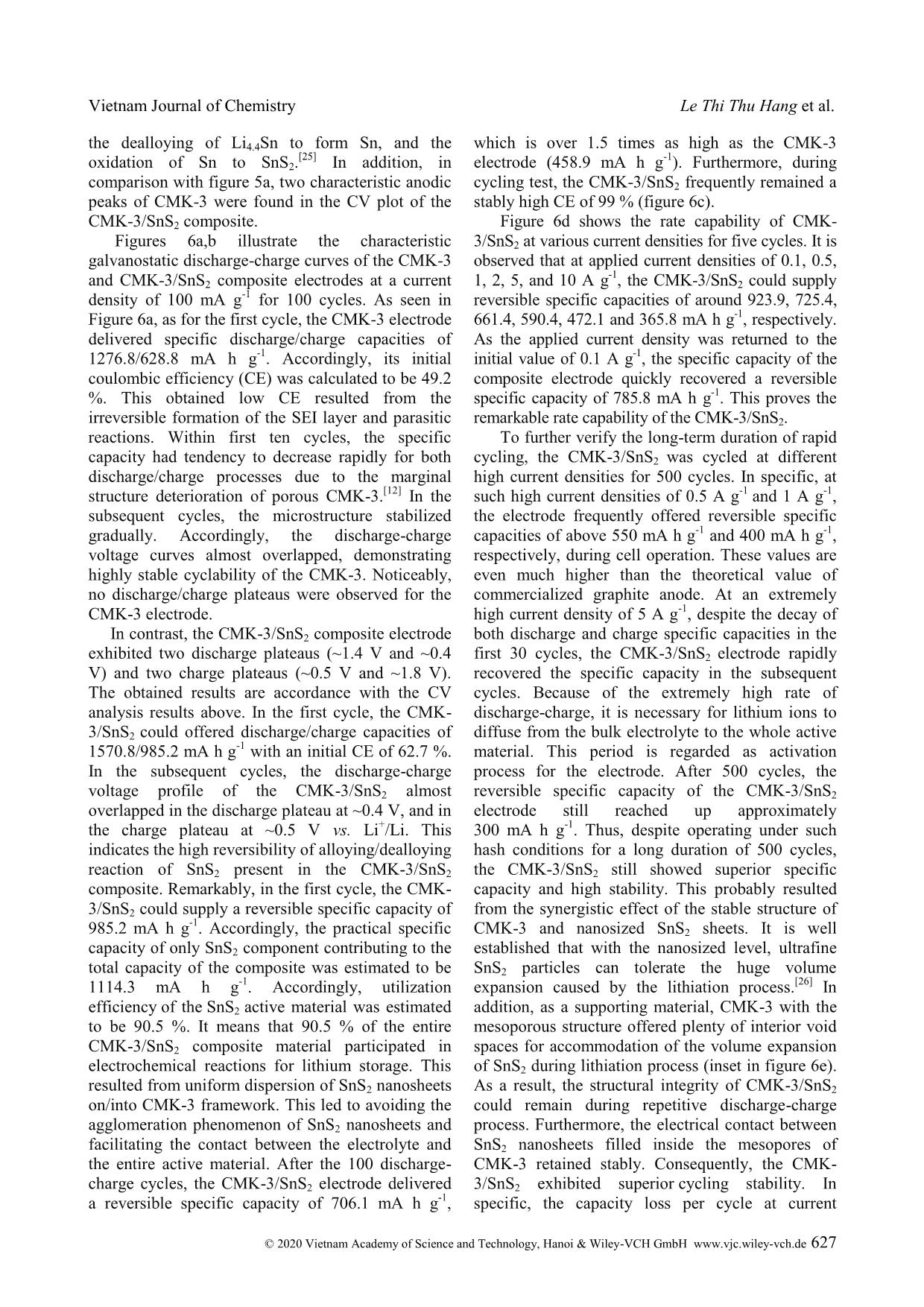
Trang 6
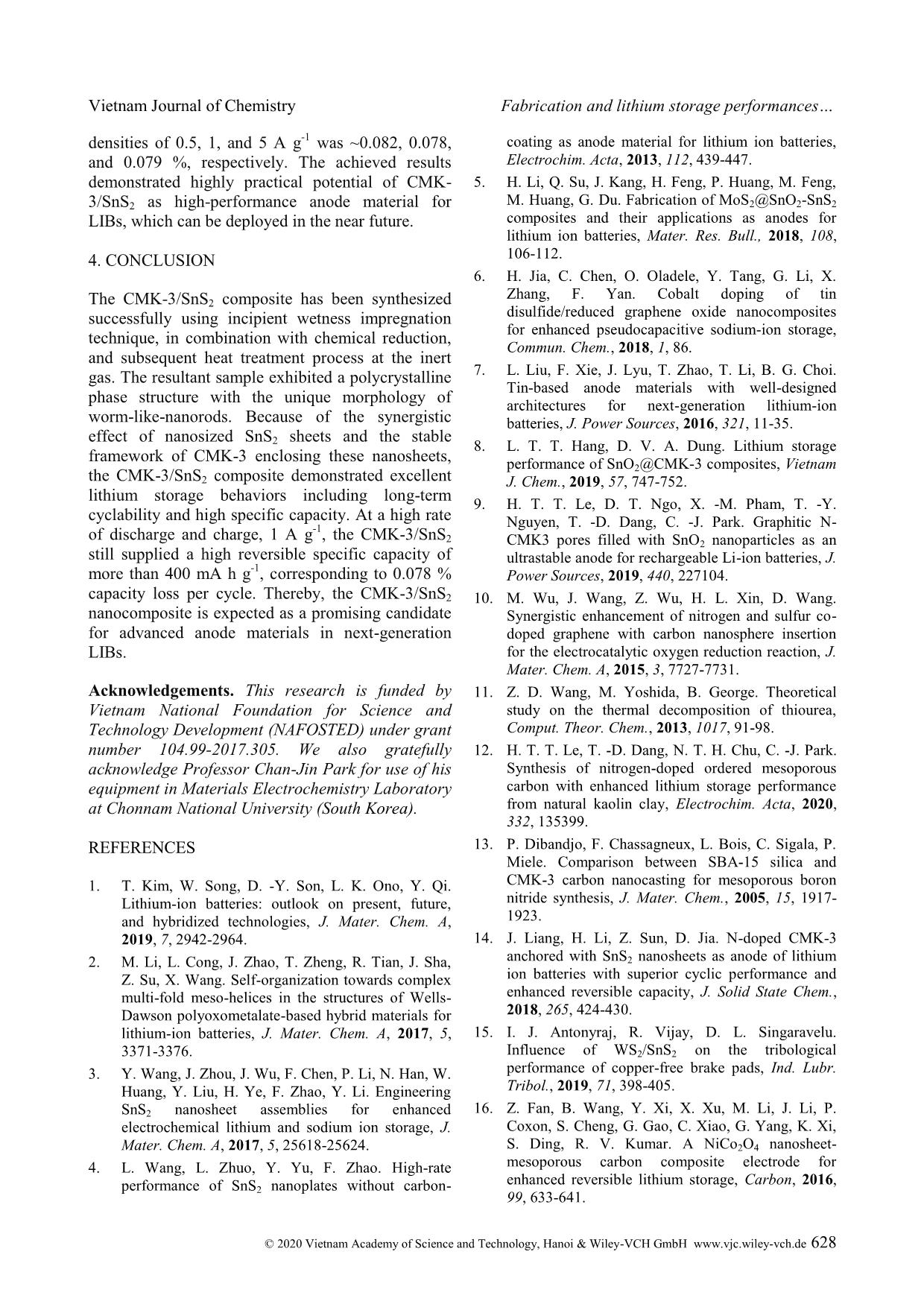
Trang 7
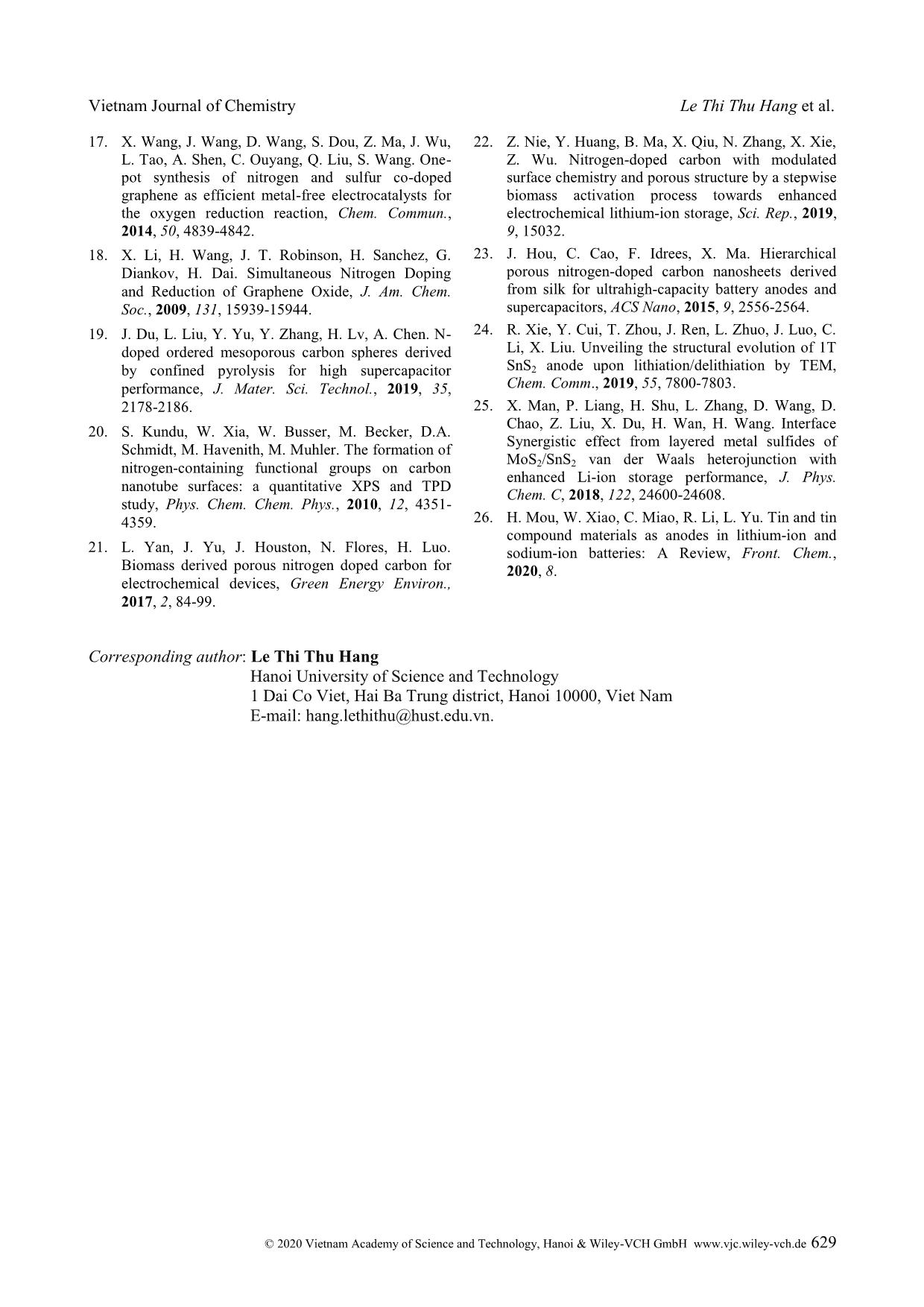
Trang 8
Tóm tắt nội dung tài liệu: Fabrication and lithium storage performances of a composite of tin disulfide and ordered mesoporous carbon
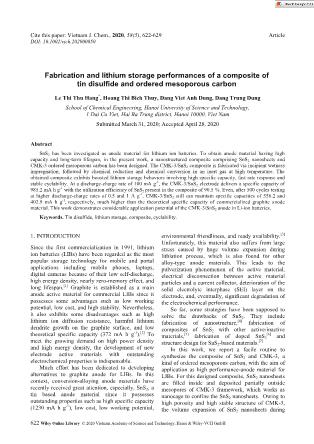
Cite this paper: Vietnam J. Chem., 2020, 58(5), 622-629 Article DOI: 10.1002/vjch.202000050 622 Wiley Online Library © 2020 Vietnam Academy of Science and Technology, Hanoi & Wiley-VCH GmbH Fabrication and lithium storage performances of a composite of tin disulfide and ordered mesoporous carbon Le Thi Thu Hang * , Hoang Thi Bich Thuy, Dang Viet Anh Dung, Dang Trung Dung School of Chemical Engineering, Hanoi University of Science and Technology, 1 Dai Co Viet, Hai Ba Trung district, Hanoi 10000, Viet Nam Submitted March 31, 2020; Accepted April 28, 2020 Abstract SnS2 has been investigated as anode material for lithium ion batteries. To obtain anode material having high capacity and long-term lifespan, in the present work, a nanostructured composite comprising SnS2 nanosheets and CMK-3 ordered mesoporous carbon has been designed. The CMK-3/SnS2 composite is fabricated via incipient wetness impregnation, followed by chemical reduction and chemical conversion in an inert gas at high temperature. The obtained composite exhibits boosted lithium storage behaviors involving high specific capacity, fast rate response and stable cyclability. At a discharge-charge rate of 100 mA g -1 , the CMK-3/SnS2 electrode delivers a specific capacity of 985.2 mA h g -1 with the utilization efficiency of SnS2 present in the composite of 90.5 %. Even, after 500 cycles testing at higher discharge-charge rates of 0.5 and 1 A g -1 , CMK-3/SnS2 still can maintain specific capacities of 556.2 and 402.9 mA h g -1 , respectively, much higher than the theoretical specific capacity of commercialized graphite anode material. This work demonstrates considerable application potential of the CMK-3/SnS2 anode in Li-ion batteries. Keywords. Tin disulfide, lithium storage, composite, cyclability. 1. INTRODUCTION Since the first commercialization in 1991, lithium ion batteries (LIBs) have been regarded as the most popular storage technology for mobile and portal applications including mobile phones, laptops, digital cameras because of their low self-discharge, high energy density, nearly zero-memory effect, and long lifespan. [1] Graphite is established as a main anode active material for commercial LIBs since it possesses some advantages such as low working potential, low cost, and high stability. Nevertheless, it also exhibits some disadvantages such as high lithium ion diffusion resistance, harmful lithium dendrite growth on the graphite surface, and low theoretical specific capacity (372 mA h g -1 ). [2] To meet the growing demand on high power density and high energy density, the development of new electrode active materials with outstanding electrochemical properties is indispensable. Much effort has been dedicated to developing alternatives to graphite anode for LIBs. In this context, conversion-alloying anode materials have recently received great attention, especially, SnS2, a tin based anode material since it possesses outstanding properties such as high specific capacity (1230 mA h g -1 ), low cost, low working potential, environmental friendliness, and ready availability. [3] Unfortunately, this material also suffers from large stress caused by huge volume expansion during lithiation process, which is also found for other alloy-type anode materials. This leads to the pulverization phenomenon of the active material, electrical disconnection between active material particles and a current collector, deterioration of the solid electrolyte interphase (SEI) layer on the electrode, and, eventually, significant degradation of the electrochemical performance. So far, some strategies have been supposed to solve the drawbacks of SnS2. They include fabrication of nanostructure, [4] fabrication of composites of SnS2 with other active/inactive materials, [5] fabrication of doped SnS2 [6] and structure design for SnS2-based materials. [7] In this work, we report a facile routine to synthesize the composite of SnS2 and CMK-3, a kind of ordered mesoporous carbon, with the aim of application as high performance-anode material for LIBs. For this designed composite, SnS2 nanosheets are filled inside and deposited partially outside mesopores of CMK-3 framework, which works as nanocage to confine the SnS2 nanosheets. Owing to high porosity and high stable structure of CMK-3, the volume expansion of SnS2 nanosheets during Vietnam Journal of Chemistry Le Thi Thu Hang et al. © 2020 Vietnam Academy of Science and Technology, Hanoi & Wiley-VCH GmbH www.vjc.wiley-vch.de 623 lithiation process can be alleviated. Accordingly, the composite exhibits remarkable lithium storage behaviors in term of cyclability, rate capability, and capacity. 2. MATERIALS AND METHODS 2.1. Synthesis of CMK-3/SnS2 composite CMK-3/SnS2 composite was fabricated via incipient wetness impregnation technique, following by heat treatment at high temperature. Where, CMK-3 as supporting material was synthesized by hard template method, which was described in the literature. [8] In detail, CMK-3 powder (0.5 g) was added in 5 mL of a 50v/50v ethanol/water mixture containing SnCl4 and ultrasonicated for 30 mins. After drying at 80 C for 5 h, the resultant powder continued to be mixed well with 2 g of NaBH4, and then storage in the humid air for 48 h. Because NaBH4 is a highly hygroscopic substance in combination with the presence of oxygen in the air, the CMK-3/SnO2 composite could be easily formed according to chemical reactions as follows: [9] SnCl4 + 4NaBH4 → Sn +4NaCl +2B2H6 +2H2 (1) Sn + O2 → SnO2 (2) After that, the CMK-3/SnO2 sample was rinsed, and dried at 100 o C, followed by a heat treatment process in nitrogen atmosphere at 350 o C for 3 h. Prior to the heat treatment, CMK-3/SnO2 powder was put into a small alumina crucible ... hese processes were irreversible and only occurred at the first discharge. Meanwhile, the rest cathodic peaks exhibited a slight shift because of the structural change of the active material after the first cycle. For the anodic scan direction, two distinct anodic peaks (0.52 V, and 1.89 V vs. Li + /Li) were recorded for the five cycles of scanning. These peaks imply Vietnam Journal of Chemistry Le Thi Thu Hang et al. © 2020 Vietnam Academy of Science and Technology, Hanoi & Wiley-VCH GmbH www.vjc.wiley-vch.de 627 the dealloying of Li4.4Sn to form Sn, and the oxidation of Sn to SnS2. [25] In addition, in comparison with figure 5a, two characteristic anodic peaks of CMK-3 were found in the CV plot of the CMK-3/SnS2 composite. Figures 6a,b illustrate the characteristic galvanostatic discharge-charge curves of the CMK-3 and CMK-3/SnS2 composite electrodes at a current density of 100 mA g -1 for 100 cycles. As seen in Figure 6a, as for the first cycle, the CMK-3 electrode delivered specific discharge/charge capacities of 1276.8/628.8 mA h g -1 . Accordingly, its initial coulombic efficiency (CE) was calculated to be 49.2 %. This obtained low CE resulted from the irreversible formation of the SEI layer and parasitic reactions. Within first ten cycles, the specific capacity had tendency to decrease rapidly for both discharge/charge processes due to the marginal structure deterioration of porous CMK-3. [12] In the subsequent cycles, the microstructure stabilized gradually. Accordingly, the discharge-charge voltage curves almost overlapped, demonstrating highly stable cyclability of the CMK-3. Noticeably, no discharge/charge plateaus were observed for the CMK-3 electrode. In contrast, the CMK-3/SnS2 composite electrode exhibited two discharge plateaus (~1.4 V and ~0.4 V) and two charge plateaus (~0.5 V and ~1.8 V). The obtained results are accordance with the CV analysis results above. In the first cycle, the CMK- 3/SnS2 could offered discharge/charge capacities of 1570.8/985.2 mA h g -1 with an initial CE of 62.7 %. In the subsequent cycles, the discharge-charge voltage profile of the CMK-3/SnS2 almost overlapped in the discharge plateau at ~0.4 V, and in the charge plateau at ~0.5 V vs. Li + /Li. This indicates the high reversibility of alloying/dealloying reaction of SnS2 present in the CMK-3/SnS2 composite. Remarkably, in the first cycle, the CMK- 3/SnS2 could supply a reversible specific capacity of 985.2 mA h g -1 . Accordingly, the practical specific capacity of only SnS2 component contributing to the total capacity of the composite was estimated to be 1114.3 mA h g -1 . Accordingly, utilization efficiency of the SnS2 active material was estimated to be 90.5 %. It means that 90.5 % of the entire CMK-3/SnS2 composite material participated in electrochemical reactions for lithium storage. This resulted from uniform dispersion of SnS2 nanosheets on/into CMK-3 framework. This led to avoiding the agglomeration phenomenon of SnS2 nanosheets and facilitating the contact between the electrolyte and the entire active material. After the 100 discharge- charge cycles, the CMK-3/SnS2 electrode delivered a reversible specific capacity of 706.1 mA h g -1 , which is over 1.5 times as high as the CMK-3 electrode (458.9 mA h g -1 ). Furthermore, during cycling test, the CMK-3/SnS2 frequently remained a stably high CE of 99 % (figure 6c). Figure 6d shows the rate capability of CMK- 3/SnS2 at various current densities for five cycles. It is observed that at applied current densities of 0.1, 0.5, 1, 2, 5, and 10 A g -1 , the CMK-3/SnS2 could supply reversible specific capacities of around 923.9, 725.4, 661.4, 590.4, 472.1 and 365.8 mA h g -1 , respectively. As the applied current density was returned to the initial value of 0.1 A g -1 , the specific capacity of the composite electrode quickly recovered a reversible specific capacity of 785.8 mA h g -1 . This proves the remarkable rate capability of the CMK-3/SnS2. To further verify the long-term duration of rapid cycling, the CMK-3/SnS2 was cycled at different high current densities for 500 cycles. In specific, at such high current densities of 0.5 A g -1 and 1 A g -1 , the electrode frequently offered reversible specific capacities of above 550 mA h g -1 and 400 mA h g -1 , respectively, during cell operation. These values are even much higher than the theoretical value of commercialized graphite anode. At an extremely high current density of 5 A g -1 , despite the decay of both discharge and charge specific capacities in the first 30 cycles, the CMK-3/SnS2 electrode rapidly recovered the specific capacity in the subsequent cycles. Because of the extremely high rate of discharge-charge, it is necessary for lithium ions to diffuse from the bulk electrolyte to the whole active material. This period is regarded as activation process for the electrode. After 500 cycles, the reversible specific capacity of the CMK-3/SnS2 electrode still reached up approximately 300 mA h g -1 . Thus, despite operating under such hash conditions for a long duration of 500 cycles, the CMK-3/SnS2 still showed superior specific capacity and high stability. This probably resulted from the synergistic effect of the stable structure of CMK-3 and nanosized SnS2 sheets. It is well established that with the nanosized level, ultrafine SnS2 particles can tolerate the huge volume expansion caused by the lithiation process. [26] In addition, as a supporting material, CMK-3 with the mesoporous structure offered plenty of interior void spaces for accommodation of the volume expansion of SnS2 during lithiation process (inset in figure 6e). As a result, the structural integrity of CMK-3/SnS2 could remain during repetitive discharge-charge process. Furthermore, the electrical contact between SnS2 nanosheets filled inside the mesopores of CMK-3 retained stably. Consequently, the CMK- 3/SnS2 exhibited superior cycling stability. In specific, the capacity loss per cycle at current Vietnam Journal of Chemistry Fabrication and lithium storage performances © 2020 Vietnam Academy of Science and Technology, Hanoi & Wiley-VCH GmbH www.vjc.wiley-vch.de 628 densities of 0.5, 1, and 5 A g -1 was ~0.082, 0.078, and 0.079 %, respectively. The achieved results demonstrated highly practical potential of CMK- 3/SnS2 as high-performance anode material for LIBs, which can be deployed in the near future. 4. CONCLUSION The CMK-3/SnS2 composite has been synthesized successfully using incipient wetness impregnation technique, in combination with chemical reduction, and subsequent heat treatment process at the inert gas. The resultant sample exhibited a polycrystalline phase structure with the unique morphology of worm-like-nanorods. Because of the synergistic effect of nanosized SnS2 sheets and the stable framework of CMK-3 enclosing these nanosheets, the CMK-3/SnS2 composite demonstrated excellent lithium storage behaviors including long-term cyclability and high specific capacity. At a high rate of discharge and charge, 1 A g -1 , the CMK-3/SnS2 still supplied a high reversible specific capacity of more than 400 mA h g -1 , corresponding to 0.078 % capacity loss per cycle. Thereby, the CMK-3/SnS2 nanocomposite is expected as a promising candidate for advanced anode materials in next-generation LIBs. Acknowledgements. This research is funded by Vietnam National Foundation for Science and Technology Development (NAFOSTED) under grant number 104.99-2017.305. We also gratefully acknowledge Professor Chan-Jin Park for use of his equipment in Materials Electrochemistry Laboratory at Chonnam National University (South Korea). REFERENCES 1. T. Kim, W. Song, D. -Y. Son, L. K. Ono, Y. Qi. Lithium-ion batteries: outlook on present, future, and hybridized technologies, J. Mater. Chem. A, 2019, 7, 2942-2964. 2. M. Li, L. Cong, J. Zhao, T. Zheng, R. Tian, J. Sha, Z. Su, X. Wang. Self-organization towards complex multi-fold meso-helices in the structures of Wells- Dawson polyoxometalate-based hybrid materials for lithium-ion batteries, J. Mater. Chem. A, 2017, 5, 3371-3376. 3. Y. Wang, J. Zhou, J. Wu, F. Chen, P. Li, N. Han, W. Huang, Y. Liu, H. Ye, F. Zhao, Y. Li. Engineering SnS2 nanosheet assemblies for enhanced electrochemical lithium and sodium ion storage, J. Mater. Chem. A, 2017, 5, 25618-25624. 4. L. Wang, L. Zhuo, Y. Yu, F. Zhao. High-rate performance of SnS2 nanoplates without carbon- coating as anode material for lithium ion batteries, Electrochim. Acta, 2013, 112, 439-447. 5. H. Li, Q. Su, J. Kang, H. Feng, P. Huang, M. Feng, M. Huang, G. Du. Fabrication of MoS2@SnO2-SnS2 composites and their applications as anodes for lithium ion batteries, Mater. Res. Bull., 2018, 108, 106-112. 6. H. Jia, C. Chen, O. Oladele, Y. Tang, G. Li, X. Zhang, F. Yan. Cobalt doping of tin disulfide/reduced graphene oxide nanocomposites for enhanced pseudocapacitive sodium-ion storage, Commun. Chem., 2018, 1, 86. 7. L. Liu, F. Xie, J. Lyu, T. Zhao, T. Li, B. G. Choi. Tin-based anode materials with well-designed architectures for next-generation lithium-ion batteries, J. Power Sources, 2016, 321, 11-35. 8. L. T. T. Hang, D. V. A. Dung. Lithium storage performance of SnO2@CMK-3 composites, Vietnam J. Chem., 2019, 57, 747-752. 9. H. T. T. Le, D. T. Ngo, X. -M. Pham, T. -Y. Nguyen, T. -D. Dang, C. -J. Park. Graphitic N- CMK3 pores filled with SnO2 nanoparticles as an ultrastable anode for rechargeable Li-ion batteries, J. Power Sources, 2019, 440, 227104. 10. M. Wu, J. Wang, Z. Wu, H. L. Xin, D. Wang. Synergistic enhancement of nitrogen and sulfur co- doped graphene with carbon nanosphere insertion for the electrocatalytic oxygen reduction reaction, J. Mater. Chem. A, 2015, 3, 7727-7731. 11. Z. D. Wang, M. Yoshida, B. George. Theoretical study on the thermal decomposition of thiourea, Comput. Theor. Chem., 2013, 1017, 91-98. 12. H. T. T. Le, T. -D. Dang, N. T. H. Chu, C. -J. Park. Synthesis of nitrogen-doped ordered mesoporous carbon with enhanced lithium storage performance from natural kaolin clay, Electrochim. Acta, 2020, 332, 135399. 13. P. Dibandjo, F. Chassagneux, L. Bois, C. Sigala, P. Miele. Comparison between SBA-15 silica and CMK-3 carbon nanocasting for mesoporous boron nitride synthesis, J. Mater. Chem., 2005, 15, 1917- 1923. 14. J. Liang, H. Li, Z. Sun, D. Jia. N-doped CMK-3 anchored with SnS2 nanosheets as anode of lithium ion batteries with superior cyclic performance and enhanced reversible capacity, J. Solid State Chem., 2018, 265, 424-430. 15. I. J. Antonyraj, R. Vijay, D. L. Singaravelu. Influence of WS2/SnS2 on the tribological performance of copper-free brake pads, Ind. Lubr. Tribol., 2019, 71, 398-405. 16. Z. Fan, B. Wang, Y. Xi, X. Xu, M. Li, J. Li, P. Coxon, S. Cheng, G. Gao, C. Xiao, G. Yang, K. Xi, S. Ding, R. V. Kumar. A NiCo2O4 nanosheet- mesoporous carbon composite electrode for enhanced reversible lithium storage, Carbon, 2016, 99, 633-641. Vietnam Journal of Chemistry Le Thi Thu Hang et al. © 2020 Vietnam Academy of Science and Technology, Hanoi & Wiley-VCH GmbH www.vjc.wiley-vch.de 629 17. X. Wang, J. Wang, D. Wang, S. Dou, Z. Ma, J. Wu, L. Tao, A. Shen, C. Ouyang, Q. Liu, S. Wang. One- pot synthesis of nitrogen and sulfur co-doped graphene as efficient metal-free electrocatalysts for the oxygen reduction reaction, Chem. Commun., 2014, 50, 4839-4842. 18. X. Li, H. Wang, J. T. Robinson, H. Sanchez, G. Diankov, H. Dai. Simultaneous Nitrogen Doping and Reduction of Graphene Oxide, J. Am. Chem. Soc., 2009, 131, 15939-15944. 19. J. Du, L. Liu, Y. Yu, Y. Zhang, H. Lv, A. Chen. N- doped ordered mesoporous carbon spheres derived by confined pyrolysis for high supercapacitor performance, J. Mater. Sci. Technol., 2019, 35, 2178-2186. 20. S. Kundu, W. Xia, W. Busser, M. Becker, D.A. Schmidt, M. Havenith, M. Muhler. The formation of nitrogen-containing functional groups on carbon nanotube surfaces: a quantitative XPS and TPD study, Phys. Chem. Chem. Phys., 2010, 12, 4351- 4359. 21. L. Yan, J. Yu, J. Houston, N. Flores, H. Luo. Biomass derived porous nitrogen doped carbon for electrochemical devices, Green Energy Environ., 2017, 2, 84-99. 22. Z. Nie, Y. Huang, B. Ma, X. Qiu, N. Zhang, X. Xie, Z. Wu. Nitrogen-doped carbon with modulated surface chemistry and porous structure by a stepwise biomass activation process towards enhanced electrochemical lithium-ion storage, Sci. Rep., 2019, 9, 15032. 23. J. Hou, C. Cao, F. Idrees, X. Ma. Hierarchical porous nitrogen-doped carbon nanosheets derived from silk for ultrahigh-capacity battery anodes and supercapacitors, ACS Nano, 2015, 9, 2556-2564. 24. R. Xie, Y. Cui, T. Zhou, J. Ren, L. Zhuo, J. Luo, C. Li, X. Liu. Unveiling the structural evolution of 1T SnS2 anode upon lithiation/delithiation by TEM, Chem. Comm., 2019, 55, 7800-7803. 25. X. Man, P. Liang, H. Shu, L. Zhang, D. Wang, D. Chao, Z. Liu, X. Du, H. Wan, H. Wang. Interface Synergistic effect from layered metal sulfides of MoS2/SnS2 van der Waals heterojunction with enhanced Li-ion storage performance, J. Phys. Chem. C, 2018, 122, 24600-24608. 26. H. Mou, W. Xiao, C. Miao, R. Li, L. Yu. Tin and tin compound materials as anodes in lithium-ion and sodium-ion batteries: A Review, Front. Chem., 2020, 8. Corresponding author: Le Thi Thu Hang Hanoi University of Science and Technology 1 Dai Co Viet, Hai Ba Trung district, Hanoi 10000, Viet Nam E-mail: hang.lethithu@hust.edu.vn.
File đính kèm:
fabrication_and_lithium_storage_performances_of_a_composite.pdf