Effect of Ionospheric Scintillation on Precise Positioning Solutions in Vietnam
The relative positioning applications can reach cm-level accuracy of positioning due to the use the very precise
but ambiguous carrier phase observations instead of pseudorange ones used in conventional absolute
positioning approach. These applications, however, are very sensitive to the irregular variation and
disturbances in the ionospheric delay. Vietnam locates at a low-latitude region near the equator where having
many irregular variations in ionosphere. In addition, here is one of the most affected region if any scintillation
occurs. In this study, we first propose a software-based receiver to detect the ionospheric scintillation in
Vietnam. After detecting the scintillation, we investigate the impact of the scintillation index to the precise
positioning solutions. For scintillation detection, we compute 𝜎𝜎𝜙𝜙, a parameter to quantify the scintillation index.
To investigate the effect of scintillation, we compare the results of RTK solutions in two scenarios: no
scintillation and scintillation. Hopefully, these results will contribute valued information to GNSS research
community in Vietnam.
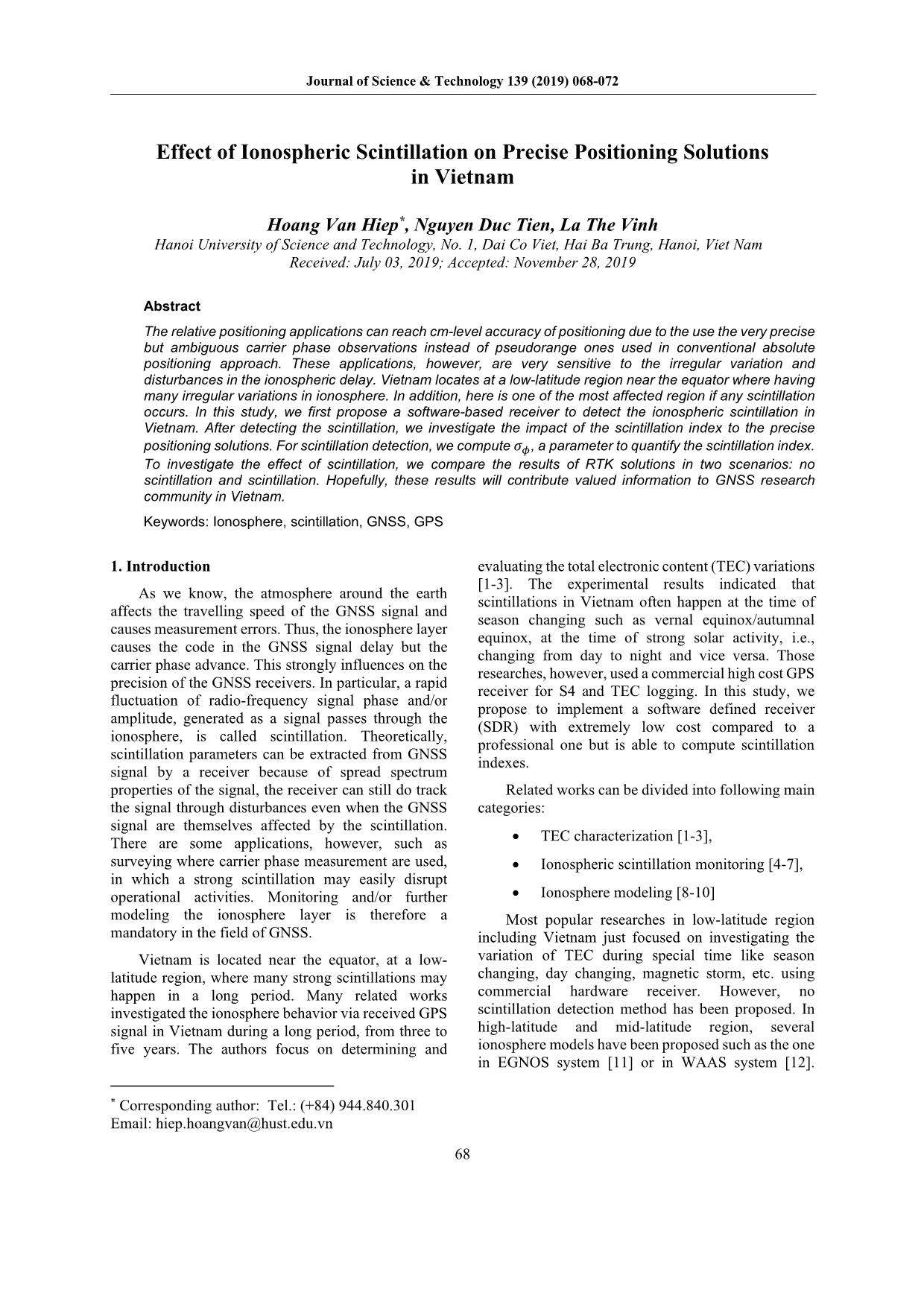
Trang 1
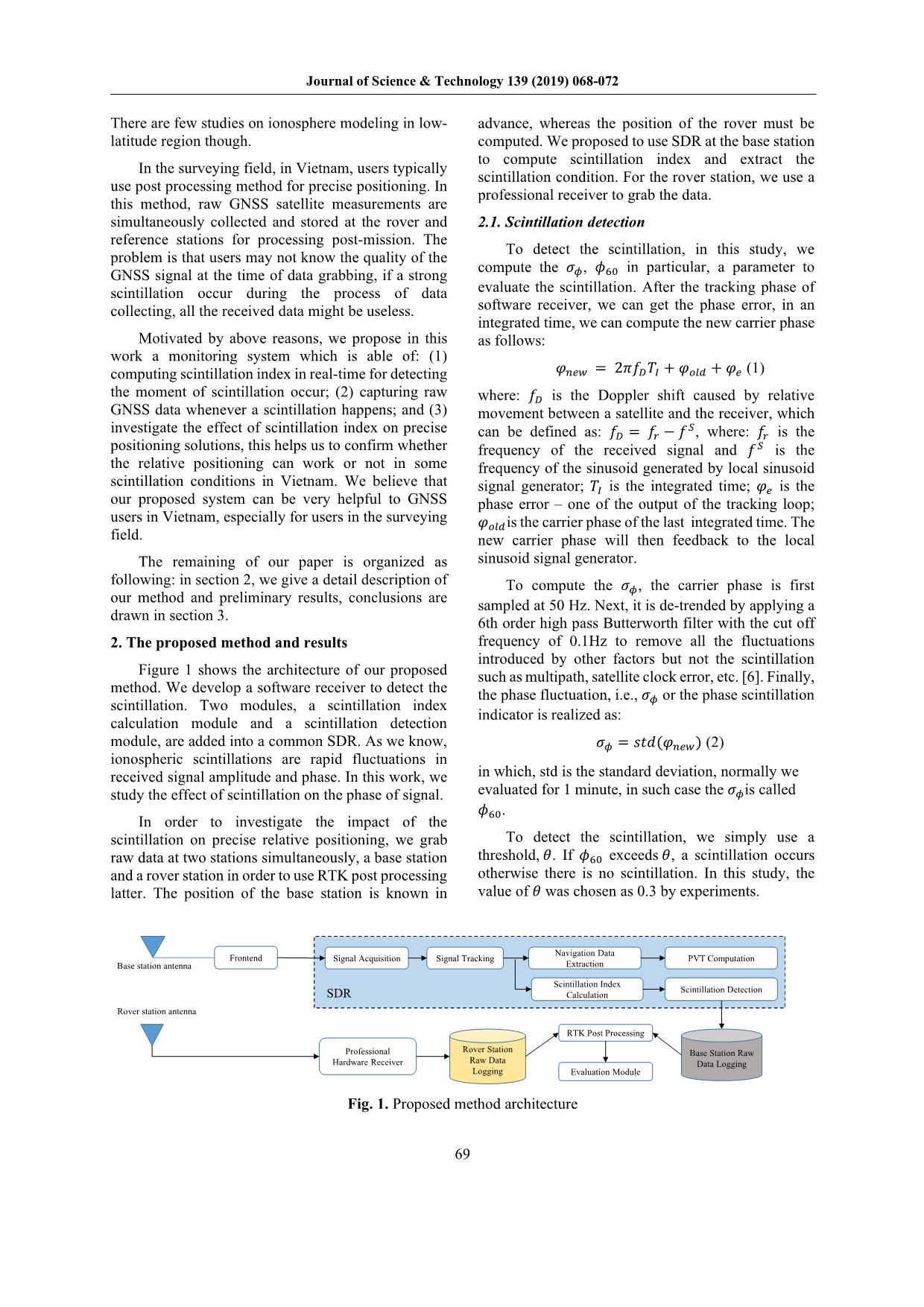
Trang 2
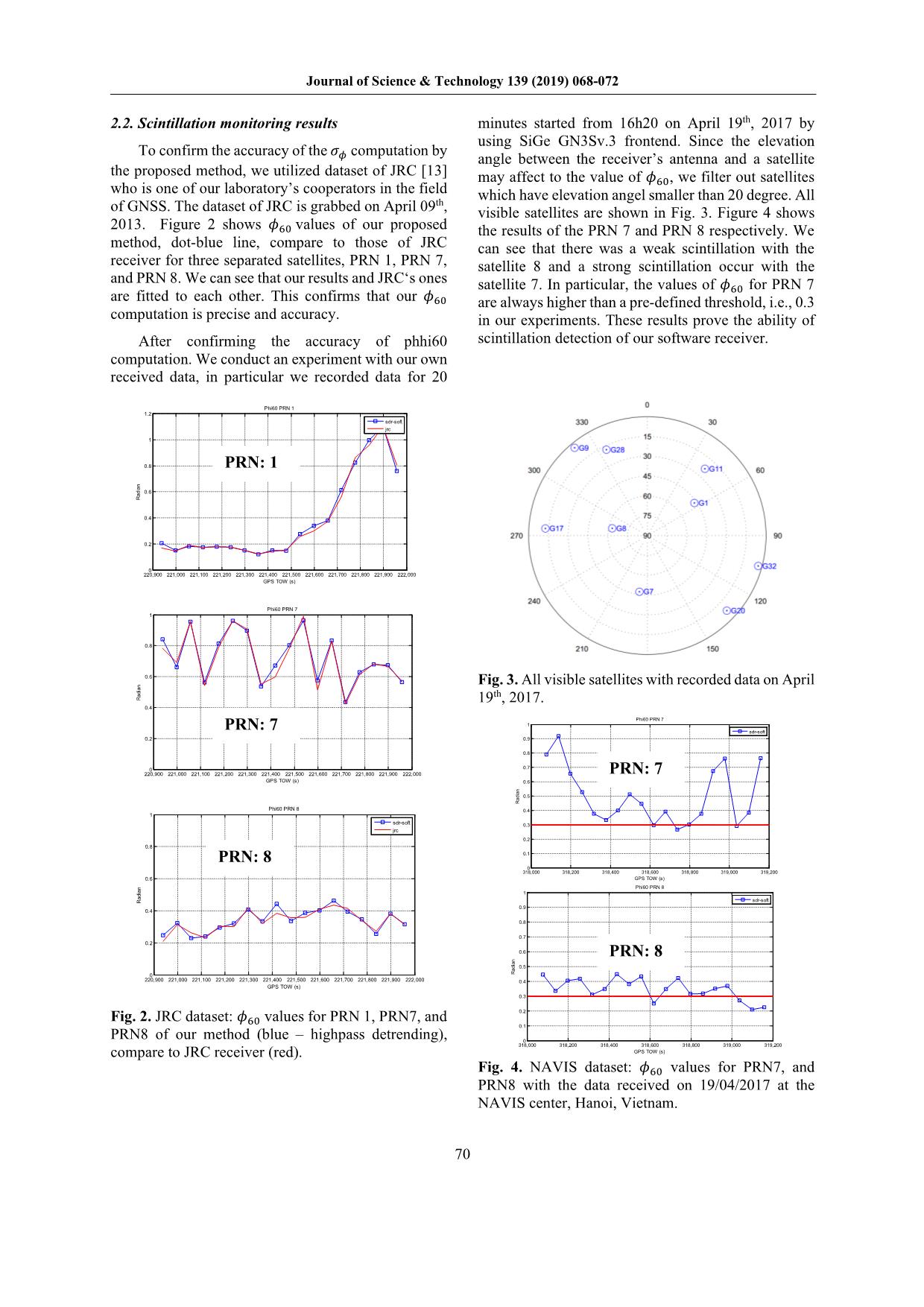
Trang 3
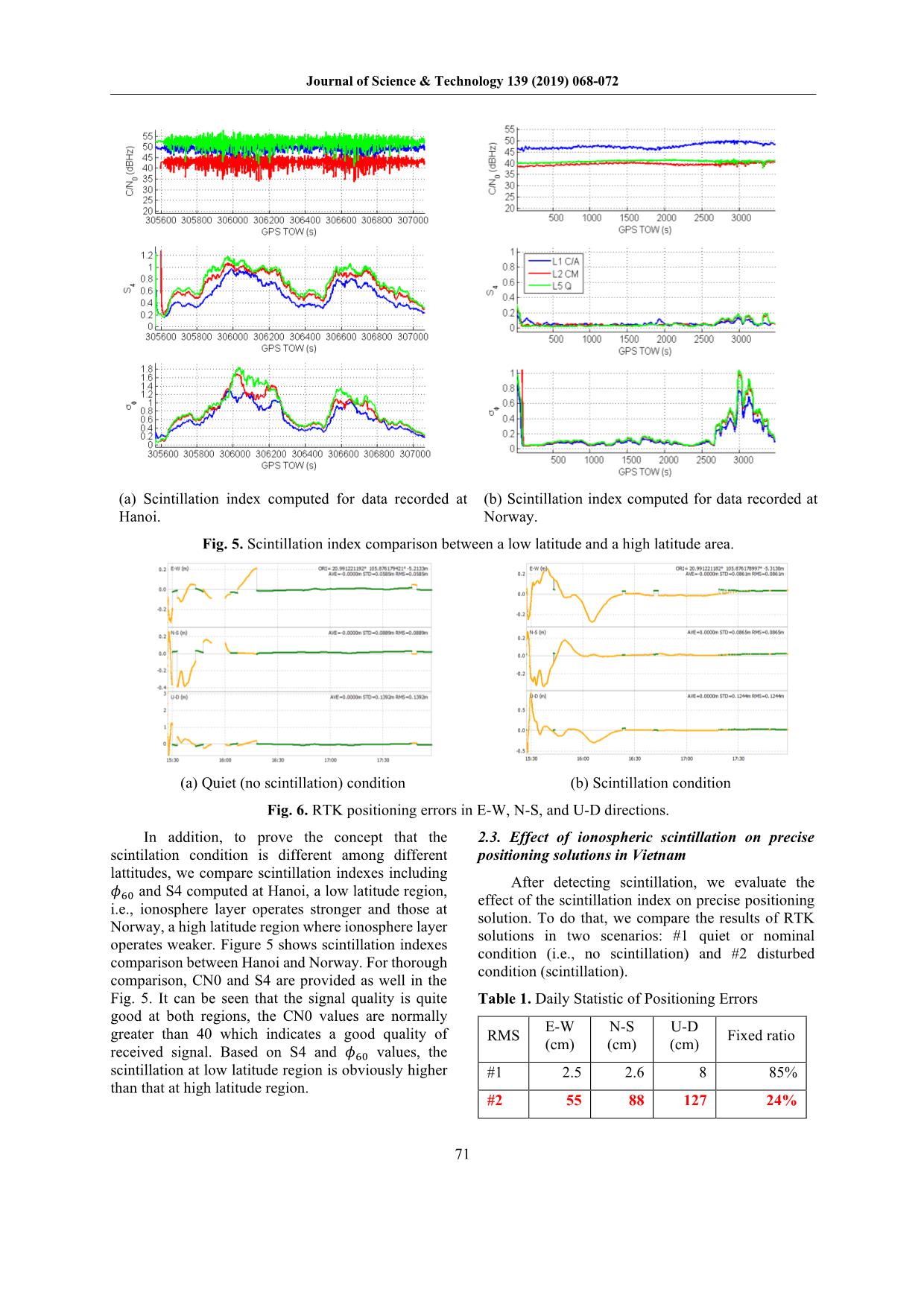
Trang 4
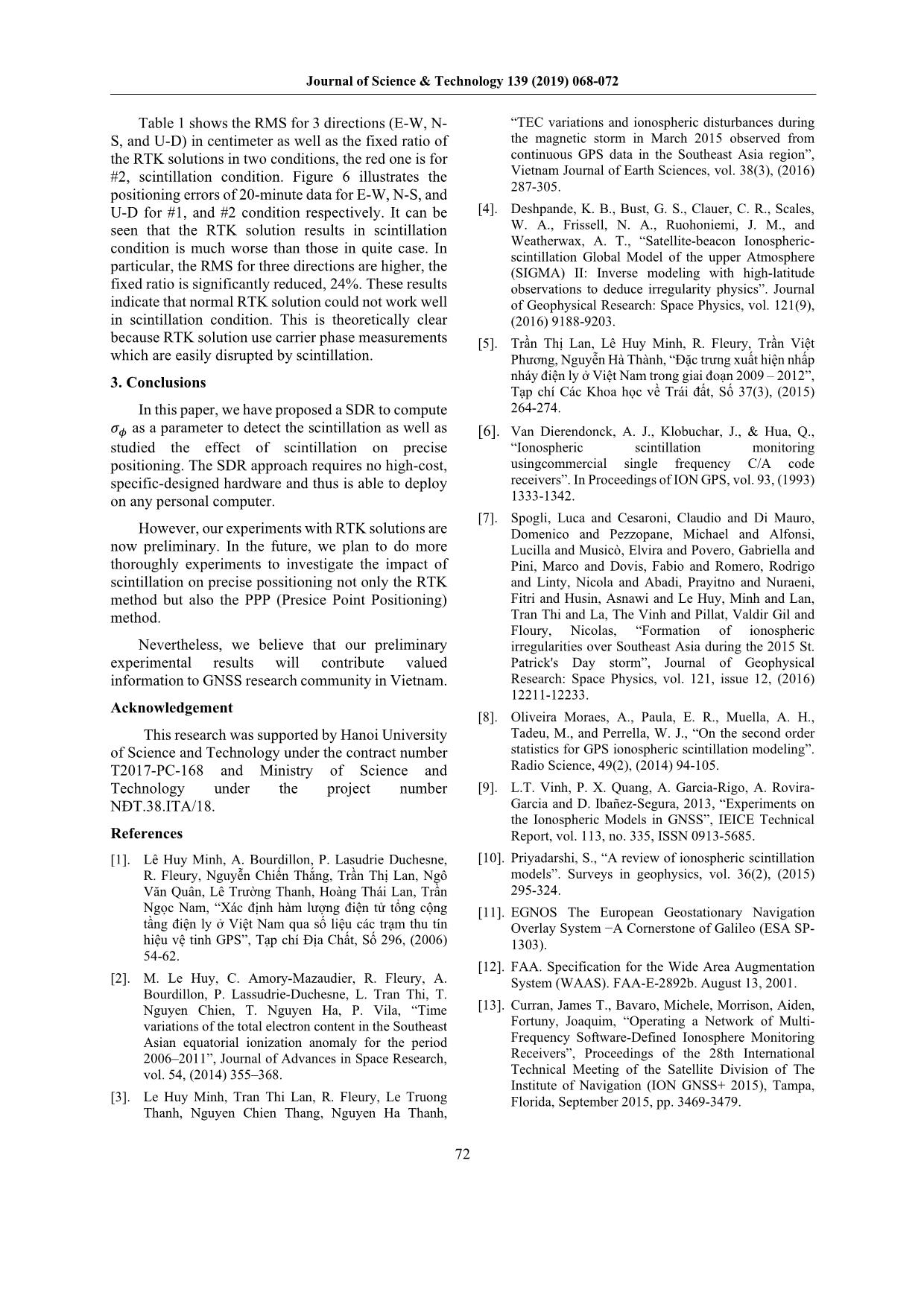
Trang 5
Tóm tắt nội dung tài liệu: Effect of Ionospheric Scintillation on Precise Positioning Solutions in Vietnam
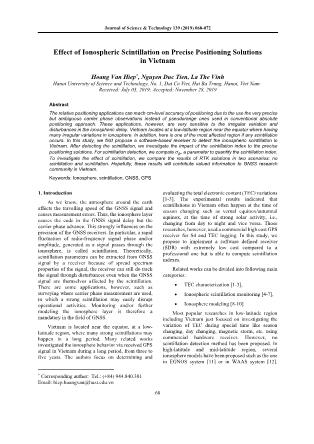
Journal of Science & Technology 139 (2019) 068-072 68 Effect of Ionospheric Scintillation on Precise Positioning Solutions in Vietnam Hoang Van Hiep*, Nguyen Duc Tien, La The Vinh Hanoi University of Science and Technology, No. 1, Dai Co Viet, Hai Ba Trung, Hanoi, Viet Nam Received: July 03, 2019; Accepted: November 28, 2019 Abstract The relative positioning applications can reach cm-level accuracy of positioning due to the use the very precise but ambiguous carrier phase observations instead of pseudorange ones used in conventional absolute positioning approach. These applications, however, are very sensitive to the irregular variation and disturbances in the ionospheric delay. Vietnam locates at a low-latitude region near the equator where having many irregular variations in ionosphere. In addition, here is one of the most affected region if any scintillation occurs. In this study, we first propose a software-based receiver to detect the ionospheric scintillation in Vietnam. After detecting the scintillation, we investigate the impact of the scintillation index to the precise positioning solutions. For scintillation detection, we compute 𝜎𝜎𝜙𝜙, a parameter to quantify the scintillation index. To investigate the effect of scintillation, we compare the results of RTK solutions in two scenarios: no scintillation and scintillation. Hopefully, these results will contribute valued information to GNSS research community in Vietnam. Keywords: Ionosphere, scintillation, GNSS, GPS 1. Introduction1 As we know, the atmosphere around the earth affects the travelling speed of the GNSS signal and causes measurement errors. Thus, the ionosphere layer causes the code in the GNSS signal delay but the carrier phase advance. This strongly influences on the precision of the GNSS receivers. In particular, a rapid fluctuation of radio-frequency signal phase and/or amplitude, generated as a signal passes through the ionosphere, is called scintillation. Theoretically, scintillation parameters can be extracted from GNSS signal by a receiver because of spread spectrum properties of the signal, the receiver can still do track the signal through disturbances even when the GNSS signal are themselves affected by the scintillation. There are some applications, however, such as surveying where carrier phase measurement are used, in which a strong scintillation may easily disrupt operational activities. Monitoring and/or further modeling the ionosphere layer is therefore a mandatory in the field of GNSS. Vietnam is located near the equator, at a low- latitude region, where many strong scintillations may happen in a long period. Many related works investigated the ionosphere behavior via received GPS signal in Vietnam during a long period, from three to five years. The authors focus on determining and * Corresponding author: Tel.: (+84) 944.840.301 Email: hiep.hoangvan@hust.edu.vn evaluating the total electronic content (TEC) variations [1-3]. The experimental results indicated that scintillations in Vietnam often happen at the time of season changing such as vernal equinox/autumnal equinox, at the time of strong solar activity, i.e., changing from day to night and vice versa. Those researches, however, used a commercial high cost GPS receiver for S4 and TEC logging. In this study, we propose to implement a software defined receiver (SDR) with extremely low cost compared to a professional one but is able to compute scintillation indexes. Related works can be divided into following main categories: • TEC characterization [1-3], • Ionospheric scintillation monitoring [4-7], • Ionosphere modeling [8-10] Most popular researches in low-latitude region including Vietnam just focused on investigating the variation of TEC during special time like season changing, day changing, magnetic storm, etc. using commercial hardware receiver. However, no scintillation detection method has been proposed. In high-latitude and mid-latitude region, several ionosphere models have been proposed such as the one in EGNOS system [11] or in WAAS system [12]. Journal of Science & Technology 139 (2019) 068-072 69 There are few studies on ionosphere modeling in low- latitude region though. In the surveying field, in Vietnam, users typically use post processing method for precise positioning. In this method, raw GNSS satellite measurements are simultaneously collected and stored at the rover and reference stations for processing post-mission. The problem is that users may not know the quality of the GNSS signal at the time of data grabbing, if a strong scintillation occur during the process of data collecting, all the received data might be useless. Motivated by above reasons, we propose in this work a monitoring system which is able of: (1) computing scintillation index in real-time for detecting the moment of scintillation occur; (2) capturing raw GNSS data whenever a scintillation happens; and (3) investigate the effect of scintillation index on precise positioning solutions, this helps us to confirm whether the relative positioning can work or not in some scintillation conditions in Vietnam. We believe that our proposed system can be very helpful to GNSS users in Vietnam, especially for users in the surveying field. The remaining of our paper is organized as following: in section 2, we give a detail description of our method and preliminary results, conclusions are drawn in section 3. 2. The proposed method and results Figure 1 shows the architecture of our proposed method. We develop a software receiver to detect the scintillation. Two modules, a scintillation index calculation module and a scintillation detection module, are added into a common SDR. As we know, ionospheric scintillations are rapid fluctuations in received signal amplitude and phase. In this work, we study the effect of scintillation on the phase of signal. In order to investigate the impact of the scintillation on precise relative positioning, we grab raw data at two stations simultaneously, a base station and a rover station in order to use RTK post processing latter. The position of the base station is known in advance, whereas the position of the rover must be computed. We proposed to use SDR at the base station to compute scintillation index and extract the scintillation condition. For the rover station, we use a professional receiver to grab the data. 2.1. Scintillation detection To detect the scintillation, in this study, we compute the 𝜎𝜎𝜙𝜙, 𝜙𝜙60 in particular, a parameter to evaluate the scintillation. After the tracking phase of software receiver, we can get the phase error, in an integrated time, we can compute the new carrier phase as follows: 𝜑𝜑𝑛𝑛𝑛𝑛𝑛𝑛 = 2𝜋𝜋𝑓𝑓𝐷𝐷𝑇𝑇𝐼𝐼 + 𝜑𝜑𝑜𝑜𝑜𝑜𝑜𝑜 + 𝜑𝜑𝑛𝑛 (1) where: 𝑓𝑓𝐷𝐷 is the Doppler shift caused by relative movement between a satellite and the receiver, which can be defined as: 𝑓𝑓𝐷𝐷 = 𝑓𝑓𝑟𝑟 − 𝑓𝑓𝑆𝑆, where: 𝑓𝑓𝑟𝑟 is the frequency of the received signal and 𝑓𝑓𝑆𝑆 is the frequency of the sinusoid generated by local sinusoid signal generator; 𝑇𝑇𝐼𝐼 is the integrated time; 𝜑𝜑𝑛𝑛 is the phase error – one of the output of the tracking loop; 𝜑𝜑𝑜𝑜𝑜𝑜𝑜𝑜 is the carrier phase of the last integrated time. The new carrier phase will then feedback to the local sinusoid signal generator. To compute the 𝜎𝜎𝜙𝜙, the carrier phase is first sampled at 50 Hz. Next, it is de-trended by applying a 6th order high pass Butterworth filter with the cut off frequency of 0.1Hz to remove all the fluctuations introduced by other factors but not the scintillation such as multipath, satellite clock error, etc. [6]. Finally, the phase fluctuation, i.e., 𝜎𝜎𝜙𝜙 or the phase scintillation indicator is realized as: 𝜎𝜎𝜙𝜙 = 𝑠𝑠𝑠𝑠𝑠𝑠(𝜑𝜑𝑛𝑛𝑛𝑛𝑛𝑛) (2) in which, std is the standard deviation, normally we evaluated for 1 minute, in such case the 𝜎𝜎𝜙𝜙is called 𝜙𝜙60. To detect the scintillation, we simply use a threshold, 𝜃𝜃. If 𝜙𝜙60 exceeds 𝜃𝜃, a scintillation occurs otherwise there is no scintillation. In this study, the value of 𝜃𝜃 was chosen as 0.3 by experiments. Fig. 1. Proposed method architecture Frontend Signal Acquisition Signal Tracking Navigation Data Extraction PVT Computation Scintillation Index CalculationSDR Base Station Raw Data Logging Base station antenna Rover station antenna Scintillation Detection Rover Station Raw Data Logging Professional Hardware Receiver RTK Post Processing Evaluation Module Journal of Science & Technology 139 (2019) 068-072 70 2.2. Scintillation monitoring results To confirm the accuracy of the 𝜎𝜎𝜙𝜙 computation by the proposed method, we utilized dataset of JRC [13] who is one of our laboratory’s cooperators in the field of GNSS. The dataset of JRC is grabbed on April 09th, 2013. Figure 2 shows 𝜙𝜙60 values of our proposed method, dot-blue line, compare to those of JRC receiver for three separated satellites, PRN 1, PRN 7, and PRN 8. We can see that our results and JRC‘s ones are fitted to each other. This confirms that our 𝜙𝜙60 computation is precise and accuracy. After confirming the accuracy of phhi60 computation. We conduct an experiment with our own received data, in particular we recorded data for 20 minutes started from 16h20 on April 19th, 2017 by using SiGe GN3Sv.3 frontend. Since the elevation angle between the receiver’s antenna and a satellite may affect to the value of 𝜙𝜙60, we filter out satellites which have elevation angel smaller than 20 degree. All visible satellites are shown in Fig. 3. Figure 4 shows the results of the PRN 7 and PRN 8 respectively. We can see that there was a weak scintillation with the satellite 8 and a strong scintillation occur with the satellite 7. In particular, the values of 𝜙𝜙60 for PRN 7 are always higher than a pre-defined threshold, i.e., 0.3 in our experiments. These results prove the ability of scintillation detection of our software receiver. Fig. 2. JRC dataset: 𝜙𝜙60 values for PRN 1, PRN7, and PRN8 of our method (blue – highpass detrending), compare to JRC receiver (red). Fig. 3. All visible satellites with recorded data on April 19th, 2017. Fig. 4. NAVIS dataset: 𝜙𝜙60 values for PRN7, and PRN8 with the data received on 19/04/2017 at the NAVIS center, Hanoi, Vietnam. 220,900 221,000 221,100 221,200 221,300 221,400 221,500 221,600 221,700 221,800 221,900 222,000 0 0.2 0.4 0.6 0.8 1 1.2 GPS TOW (s) R ad ia n Phi60 PRN 1 sdr-soft jrc 220,900 221,000 221,100 221,200 221,300 221,400 221,500 221,600 221,700 221,800 221,900 222,000 0 0.2 0.4 0.6 0.8 1 Ra di an GPS TOW (s) Phi60 PRN 7 220,900 221,000 221,100 221,200 221,300 221,400 221,500 221,600 221,700 221,800 221,900 222,000 0 0.2 0.4 0.6 0.8 1 GPS TOW (s) R ad ia n Phi60 PRN 8 sdr-soft jrc 318,000 318,200 318,400 318,600 318,800 319,000 319,200 0 0.1 0.2 0.3 0.4 0.5 0.6 0.7 0.8 0.9 1 GPS TOW (s) R ad ia n Phi60 PRN 7 sdr-soft 318,000 318,200 318,400 318,600 318,800 319,000 319,200 0 0.1 0.2 0.3 0.4 0.5 0.6 0.7 0.8 0.9 1 GPS TOW (s) R ad ia n Phi60 PRN 8 sdr-soft PRN: 7 PRN: 8 PRN: 1 PRN: 7 PRN: 8 Journal of Science & Technology 139 (2019) 068-072 71 In addition, to prove the concept that the scintilation condition is different among different lattitudes, we compare scintillation indexes including 𝜙𝜙60 and S4 computed at Hanoi, a low latitude region, i.e., ionosphere layer operates stronger and those at Norway, a high latitude region where ionosphere layer operates weaker. Figure 5 shows scintillation indexes comparison between Hanoi and Norway. For thorough comparison, CN0 and S4 are provided as well in the Fig. 5. It can be seen that the signal quality is quite good at both regions, the CN0 values are normally greater than 40 which indicates a good quality of received signal. Based on S4 and 𝜙𝜙60 values, the scintillation at low latitude region is obviously higher than that at high latitude region. 2.3. Effect of ionospheric scintillation on precise positioning solutions in Vietnam After detecting scintillation, we evaluate the effect of the scintillation index on precise positioning solution. To do that, we compare the results of RTK solutions in two scenarios: #1 quiet or nominal condition (i.e., no scintillation) and #2 disturbed condition (scintillation). Table 1. Daily Statistic of Positioning Errors RMS E-W (cm) N-S (cm) U-D (cm) Fixed ratio #1 2.5 2.6 8 85% #2 55 88 127 24% (a) Scintillation index computed for data recorded at Hanoi. (b) Scintillation index computed for data recorded at Norway. Fig. 5. Scintillation index comparison between a low latitude and a high latitude area. (a) Quiet (no scintillation) condition (b) Scintillation condition Fig. 6. RTK positioning errors in E-W, N-S, and U-D directions. Journal of Science & Technology 139 (2019) 068-072 72 Table 1 shows the RMS for 3 directions (E-W, N- S, and U-D) in centimeter as well as the fixed ratio of the RTK solutions in two conditions, the red one is for #2, scintillation condition. Figure 6 illustrates the positioning errors of 20-minute data for E-W, N-S, and U-D for #1, and #2 condition respectively. It can be seen that the RTK solution results in scintillation condition is much worse than those in quite case. In particular, the RMS for three directions are higher, the fixed ratio is significantly reduced, 24%. These results indicate that normal RTK solution could not work well in scintillation condition. This is theoretically clear because RTK solution use carrier phase measurements which are easily disrupted by scintillation. 3. Conclusions In this paper, we have proposed a SDR to compute 𝜎𝜎𝜙𝜙 as a parameter to detect the scintillation as well as studied the effect of scintillation on precise positioning. The SDR approach requires no high-cost, specific-designed hardware and thus is able to deploy on any personal computer. However, our experiments with RTK solutions are now preliminary. In the future, we plan to do more thoroughly experiments to investigate the impact of scintillation on precise possitioning not only the RTK method but also the PPP (Presice Point Positioning) method. Nevertheless, we believe that our preliminary experimental results will contribute valued information to GNSS research community in Vietnam. Acknowledgement This research was supported by Hanoi University of Science and Technology under the contract number T2017-PC-168 and Ministry of Science and Technology under the project number NĐT.38.ITA/18. References [1]. Lê Huy Minh, A. Bourdillon, P. Lasudrie Duchesne, R. Fleury, Nguyễn Chiến Thắng, Trần Thị Lan, Ngô Văn Quân, Lê Trường Thanh, Hoàng Thái Lan, Trần Ngọc Nam, “Xác định hàm lượng điện tử tổng cộng tầng điện ly ở Việt Nam qua số liệu các trạm thu tín hiệu vệ tinh GPS”, Tạp chí Địa Chất, Số 296, (2006) 54-62. [2]. M. Le Huy, C. Amory-Mazaudier, R. Fleury, A. Bourdillon, P. Lassudrie-Duchesne, L. Tran Thi, T. Nguyen Chien, T. Nguyen Ha, P. Vila, “Time variations of the total electron content in the Southeast Asian equatorial ionization anomaly for the period 2006–2011”, Journal of Advances in Space Research, vol. 54, (2014) 355–368. [3]. Le Huy Minh, Tran Thi Lan, R. Fleury, Le Truong Thanh, Nguyen Chien Thang, Nguyen Ha Thanh, “TEC variations and ionospheric disturbances during the magnetic storm in March 2015 observed from continuous GPS data in the Southeast Asia region”, Vietnam Journal of Earth Sciences, vol. 38(3), (2016) 287-305. [4]. Deshpande, K. B., Bust, G. S., Clauer, C. R., Scales, W. A., Frissell, N. A., Ruohoniemi, J. M., and Weatherwax, A. T., “Satellite‐beacon Ionospheric‐ scintillation Global Model of the upper Atmosphere (SIGMA) II: Inverse modeling with high‐latitude observations to deduce irregularity physics”. Journal of Geophysical Research: Space Physics, vol. 121(9), (2016) 9188-9203. [5]. Trần Thị Lan, Lê Huy Minh, R. Fleury, Trần Việt Phương, Nguyễn Hà Thành, “Đặc trưng xuất hiện nhấp nháy điện ly ở Việt Nam trong giai đoạn 2009 – 2012”, Tạp chí Các Khoa học về Trái đất, Số 37(3), (2015) 264-274. [6]. Van Dierendonck, A. J., Klobuchar, J., & Hua, Q., “Ionospheric scintillation monitoring usingcommercial single frequency C/A code receivers”. In Proceedings of ION GPS, vol. 93, (1993) 1333-1342. [7]. Spogli, Luca and Cesaroni, Claudio and Di Mauro, Domenico and Pezzopane, Michael and Alfonsi, Lucilla and Musicò, Elvira and Povero, Gabriella and Pini, Marco and Dovis, Fabio and Romero, Rodrigo and Linty, Nicola and Abadi, Prayitno and Nuraeni, Fitri and Husin, Asnawi and Le Huy, Minh and Lan, Tran Thi and La, The Vinh and Pillat, Valdir Gil and Floury, Nicolas, “Formation of ionospheric irregularities over Southeast Asia during the 2015 St. Patrick's Day storm”, Journal of Geophysical Research: Space Physics, vol. 121, issue 12, (2016) 12211-12233. [8]. Oliveira Moraes, A., Paula, E. R., Muella, A. H., Tadeu, M., and Perrella, W. J., “On the second order statistics for GPS ionospheric scintillation modeling”. Radio Science, 49(2), (2014) 94-105. [9]. L.T. Vinh, P. X. Quang, A. Garcia-Rigo, A. Rovira- Garcia and D. Ibañez-Segura, 2013, “Experiments on the Ionospheric Models in GNSS”, IEICE Technical Report, vol. 113, no. 335, ISSN 0913-5685. [10]. Priyadarshi, S., “A review of ionospheric scintillation models”. Surveys in geophysics, vol. 36(2), (2015) 295-324. [11]. EGNOS The European Geostationary Navigation Overlay System −A Cornerstone of Galileo (ESA SP- 1303). [12]. FAA. Specification for the Wide Area Augmentation System (WAAS). FAA-E-2892b. August 13, 2001. [13]. Curran, James T., Bavaro, Michele, Morrison, Aiden, Fortuny, Joaquim, “Operating a Network of Multi- Frequency Software-Defined Ionosphere Monitoring Receivers”, Proceedings of the 28th International Technical Meeting of the Satellite Division of The Institute of Navigation (ION GNSS+ 2015), Tampa, Florida, September 2015, pp. 3469-3479.
File đính kèm:
effect_of_ionospheric_scintillation_on_precise_positioning_s.pdf