Structure, morphological, magnetic and optical properties of CuxMg1-xFe₂O₄ (x = 0, 0.5, 1) nano ferrites synthesized by co-precipitation method
CuxMg1.xFe2O4 nanoparticles were successfully synthesized by coprecipitation. The samples were calcined at 900 oC for 3 h and X-ray diffraction
analysis showed that Cu0.5Mg0.5Fe2O4 had a single phase cubic spinel structure,
while formation of secondary phase of Fe2O3 was observed in XRD patterns of
CuFe2O4, MgFe2O4. The saturation magnetization (Ms) of Cu0.5Mg0.5Fe2O4 is in
between the saturation magnetization values of CuFe2O4 and MgFe2O4
nanoparticles, CuFe2O4 is a ferromagnetic material, while MgFe2O4 and
Cu0.5Mg0.5Fe2O4 show superparamagnetic behavior. The synthesized spinel ferrites
were fully characterized using scanning electron microscopy (SEM), FTIR
spectroscopy, energy dispersive spectroscopy (EDS) and UV-vis spectrophotometry.
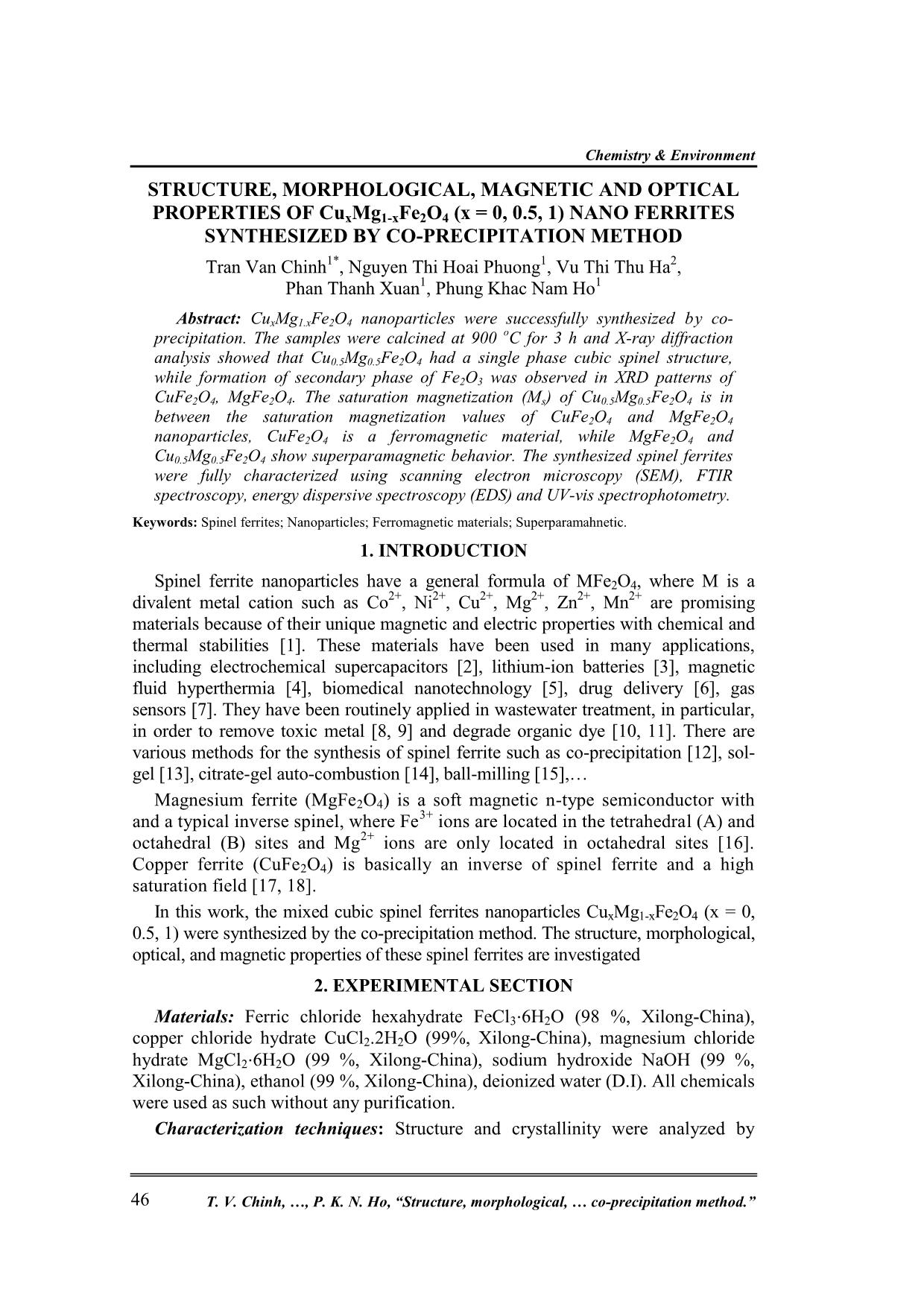
Trang 1
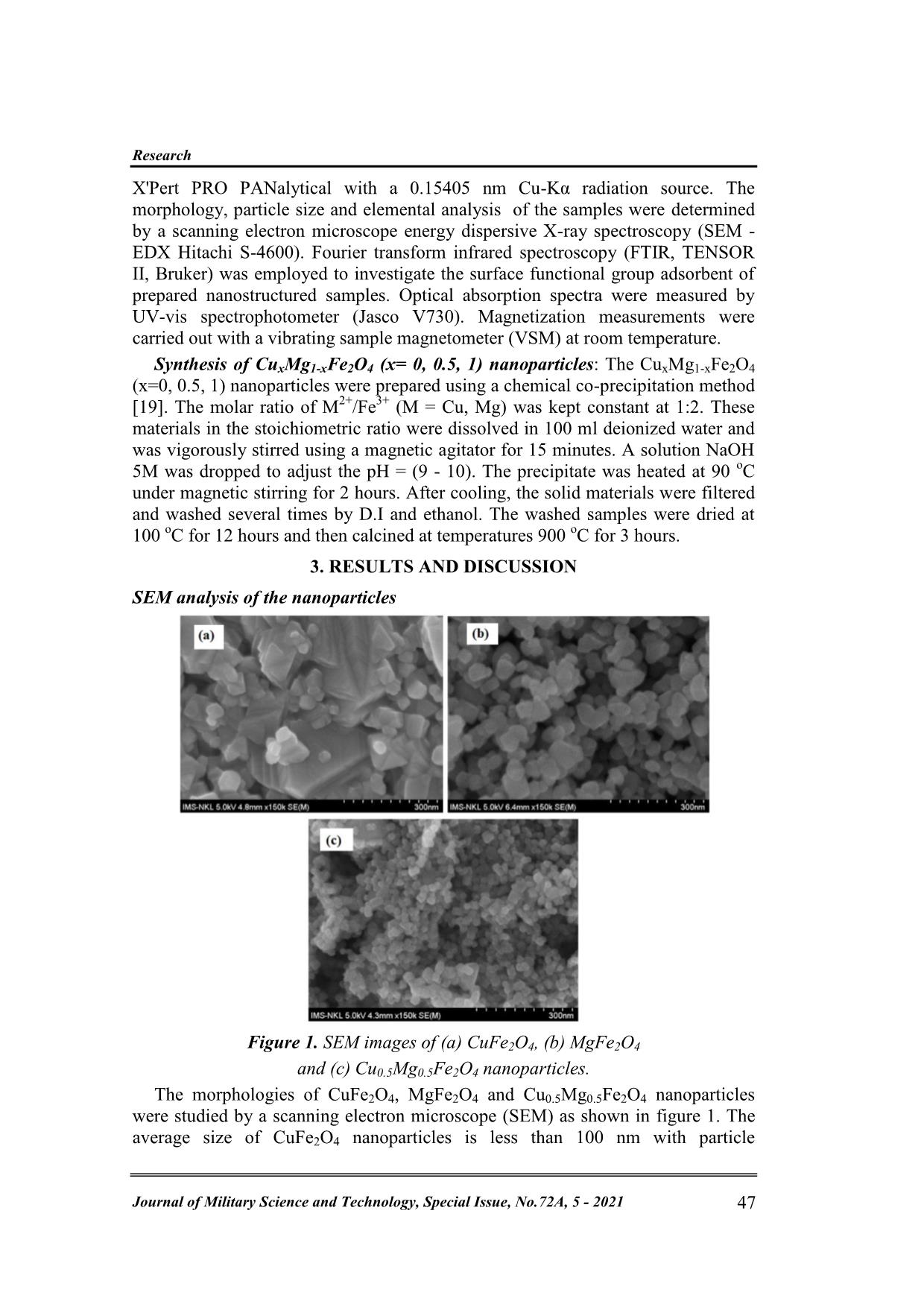
Trang 2
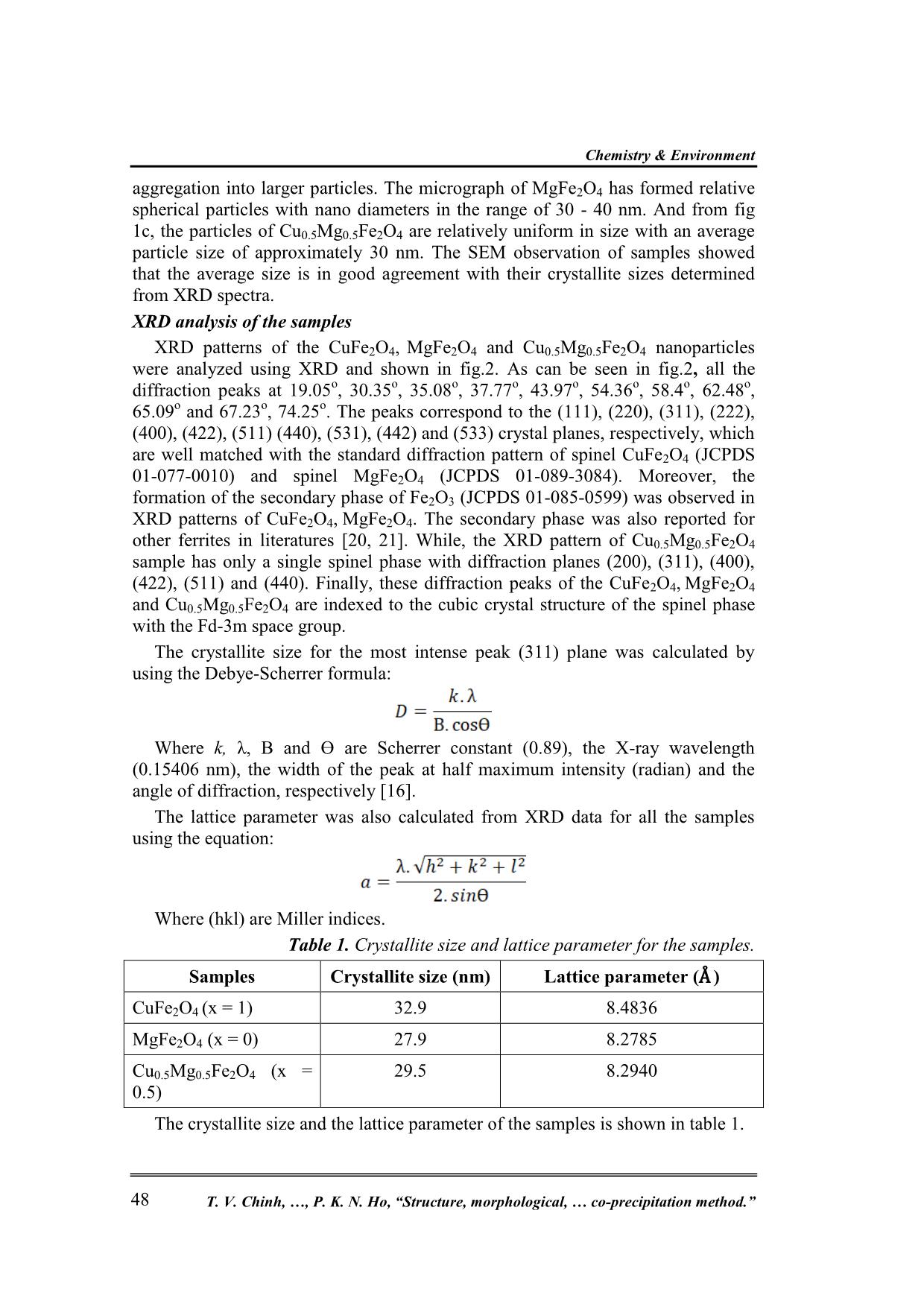
Trang 3
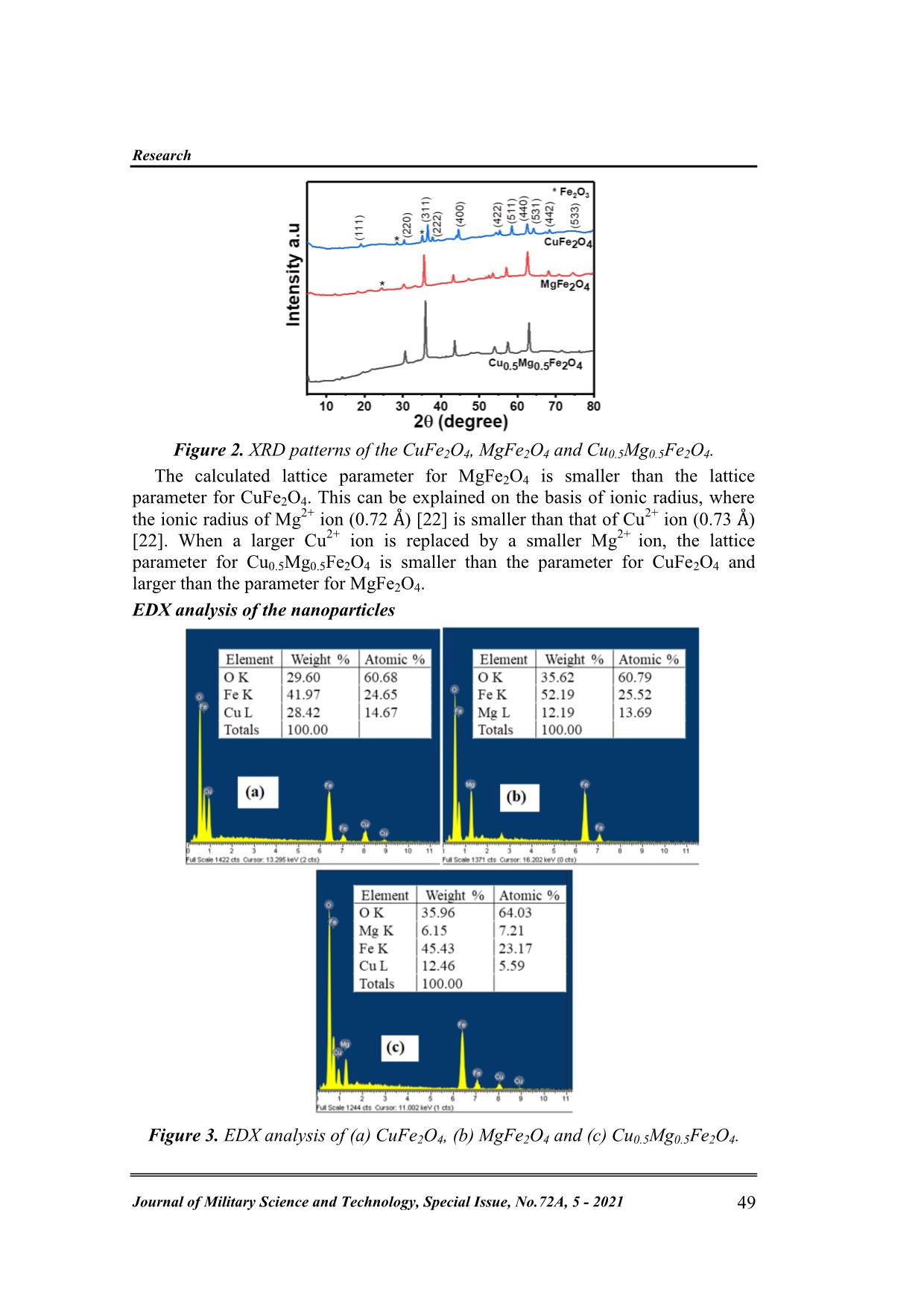
Trang 4
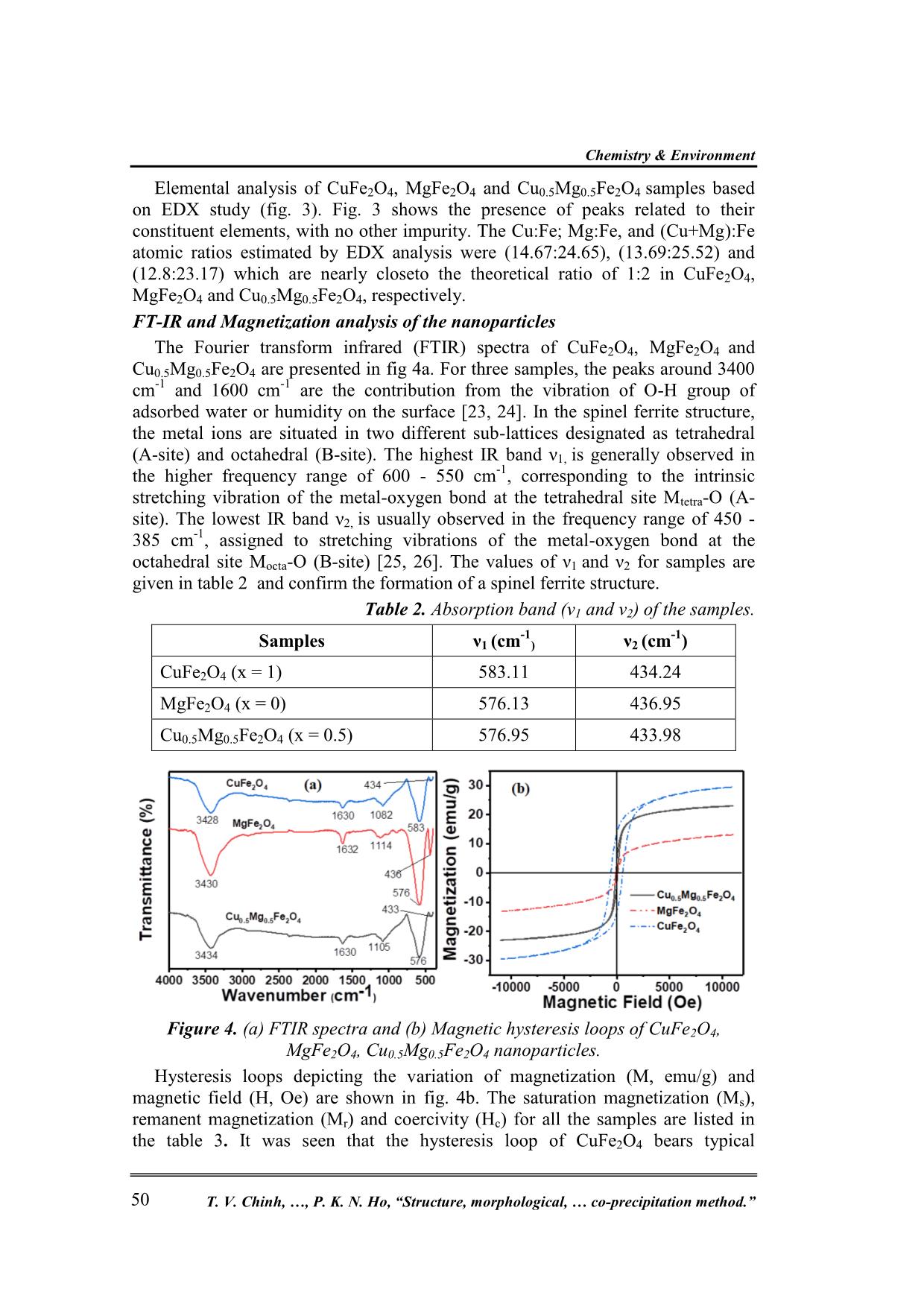
Trang 5
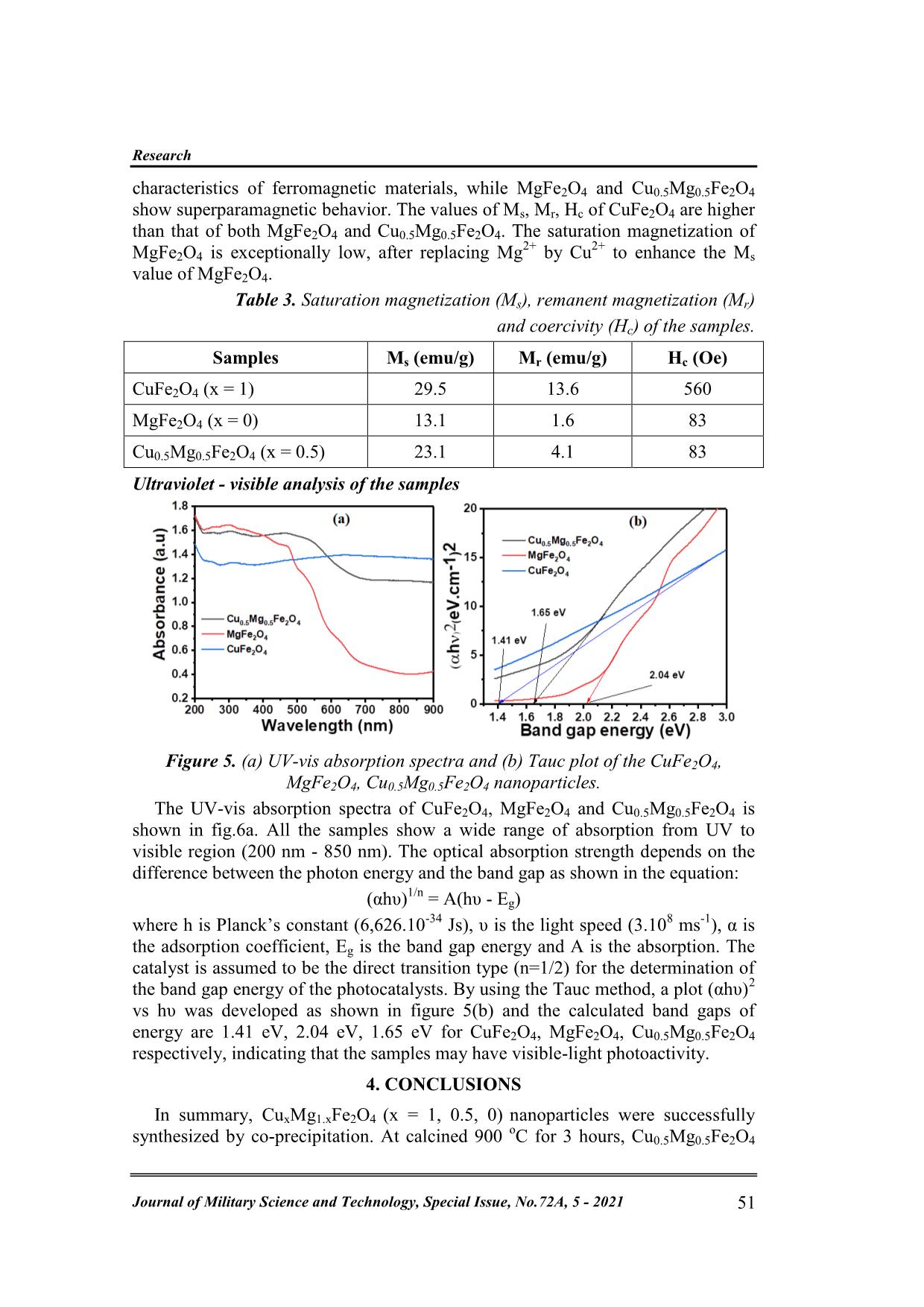
Trang 6
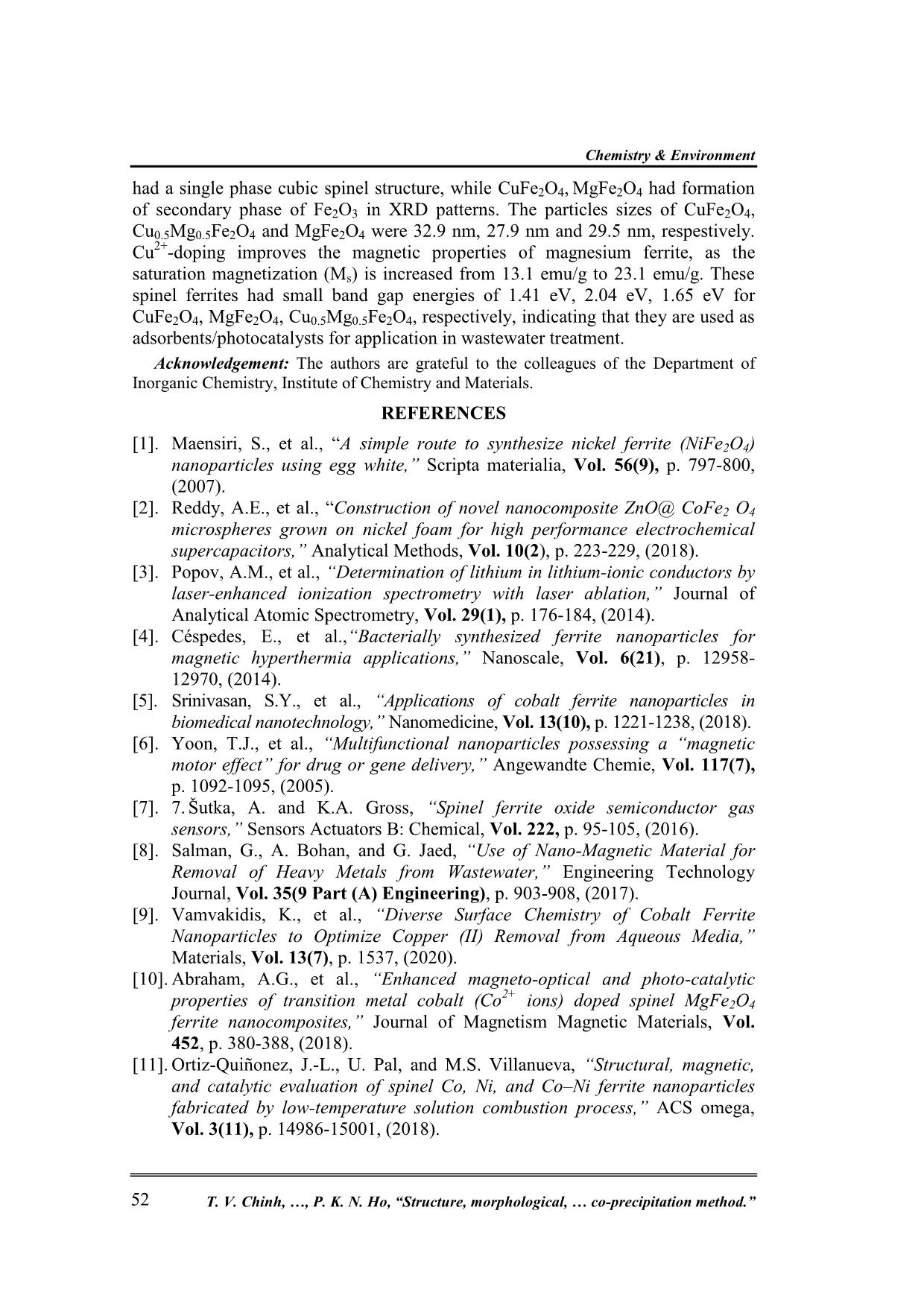
Trang 7
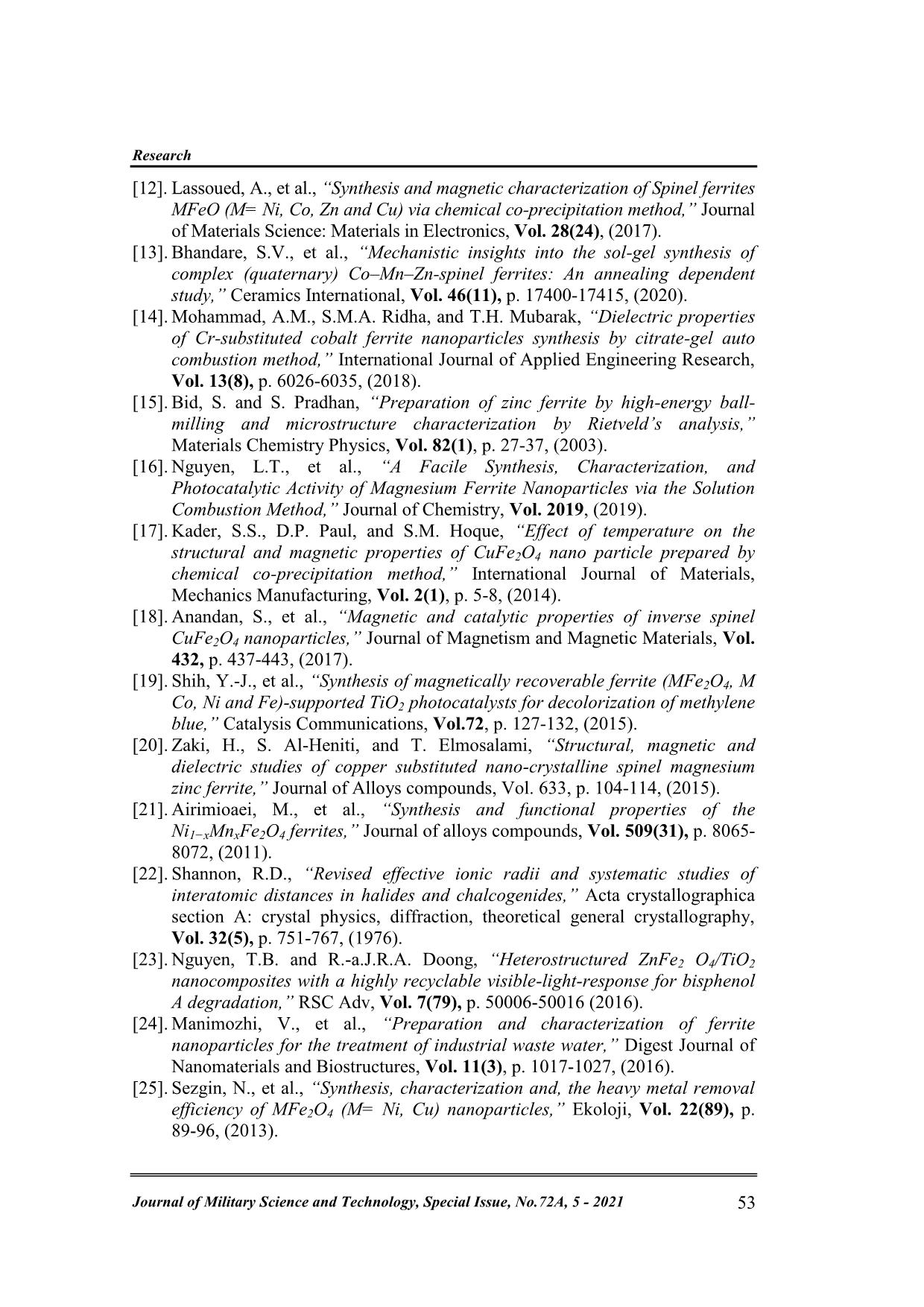
Trang 8
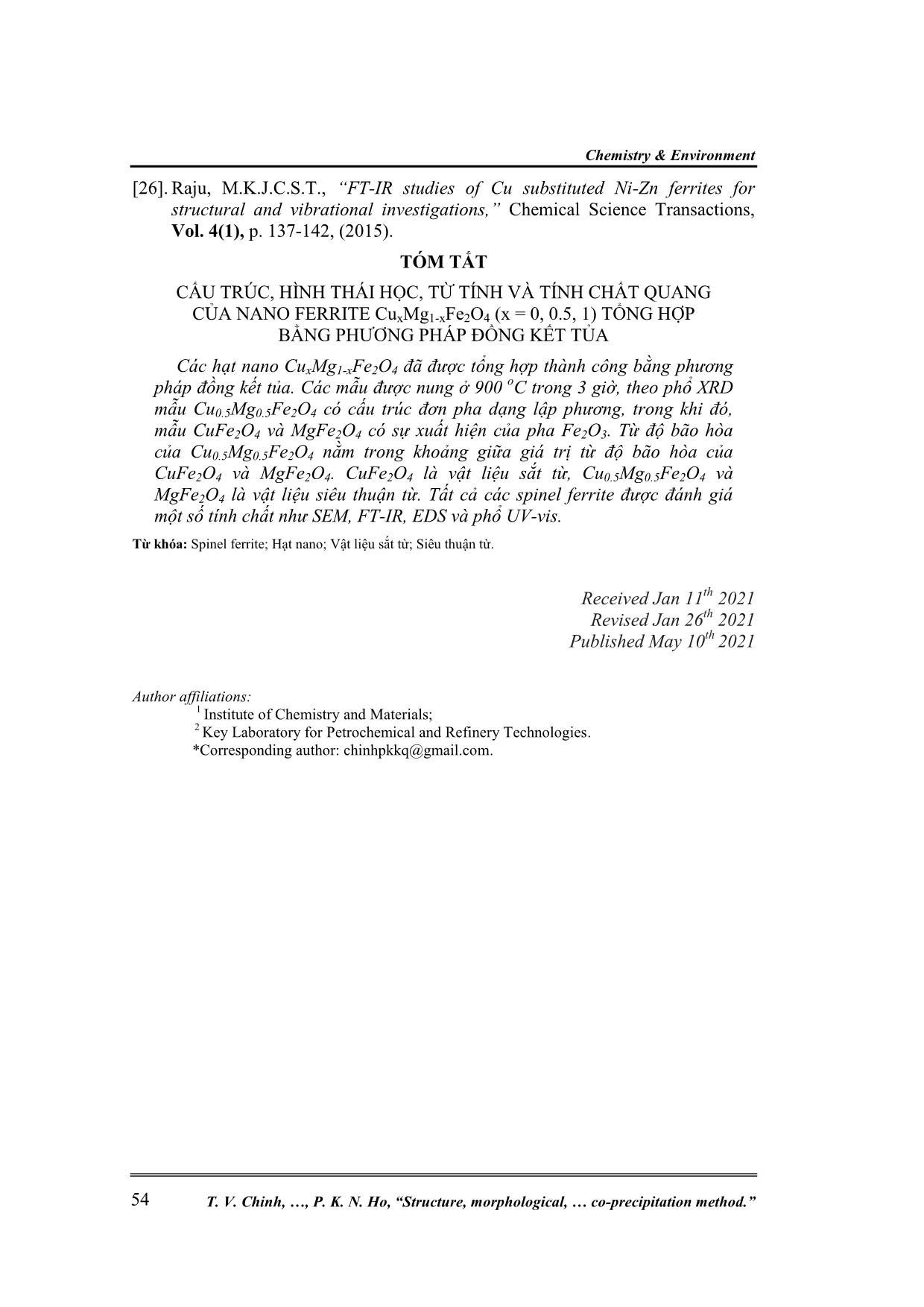
Trang 9
Tóm tắt nội dung tài liệu: Structure, morphological, magnetic and optical properties of CuxMg1-xFe₂O₄ (x = 0, 0.5, 1) nano ferrites synthesized by co-precipitation method
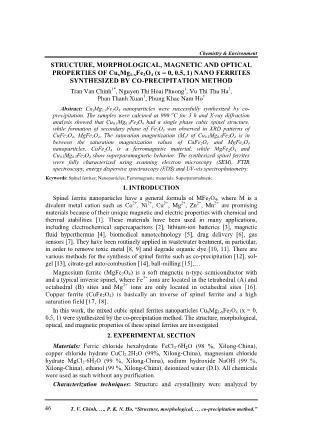
Chemistry & Environment T. V. Chinh, , P. K. N. Ho, “Structure, morphological, co-precipitation method.” 46 STRUCTURE, MORPHOLOGICAL, MAGNETIC AND OPTICAL PROPERTIES OF CuxMg1-xFe2O4 (x = 0, 0.5, 1) NANO FERRITES SYNTHESIZED BY CO-PRECIPITATION METHOD Tran Van Chinh 1* , Nguyen Thi Hoai Phuong 1 , Vu Thi Thu Ha 2 , Phan Thanh Xuan 1 , Phung Khac Nam Ho 1 Abstract: CuxMg1.xFe2O4 nanoparticles were successfully synthesized by co- precipitation. The samples were calcined at 900 o C for 3 h and X-ray diffraction analysis showed that Cu0.5Mg0.5Fe2O4 had a single phase cubic spinel structure, while formation of secondary phase of Fe2O3 was observed in XRD patterns of CuFe2O4, MgFe2O4. The saturation magnetization (Ms) of Cu0.5Mg0.5Fe2O4 is in between the saturation magnetization values of CuFe2O4 and MgFe2O4 nanoparticles, CuFe2O4 is a ferromagnetic material, while MgFe2O4 and Cu0.5Mg0.5Fe2O4 show superparamagnetic behavior. The synthesized spinel ferrites were fully characterized using scanning electron microscopy (SEM), FTIR spectroscopy, energy dispersive spectroscopy (EDS) and UV-vis spectrophotometry. Keywords: Spinel ferrites; Nanoparticles; Ferromagnetic materials; Superparamahnetic. 1. INTRODUCTION Spinel ferrite nanoparticles have a general formula of MFe2O4, where M is a divalent metal cation such as Co 2+ , Ni 2+ , Cu 2+ , Mg 2+ , Zn 2+ , Mn 2+ are promising materials because of their unique magnetic and electric properties with chemical and thermal stabilities [1]. These materials have been used in many applications, including electrochemical supercapacitors [2], lithium-ion batteries [3], magnetic fluid hyperthermia [4], biomedical nanotechnology [5], drug delivery [6], gas sensors [7]. They have been routinely applied in wastewater treatment, in particular, in order to remove toxic metal [8, 9] and degrade organic dye [10, 11]. There are various methods for the synthesis of spinel ferrite such as co-precipitation [12], sol- gel [13], citrate-gel auto-combustion [14], ball-milling [15], Magnesium ferrite (MgFe2O4) is a soft magnetic n-type semiconductor with and a typical inverse spinel, where Fe 3+ ions are located in the tetrahedral (A) and octahedral (B) sites and Mg 2+ ions are only located in octahedral sites [16]. Copper ferrite (CuFe2O4) is basically an inverse of spinel ferrite and a high saturation field [17, 18]. In this work, the mixed cubic spinel ferrites nanoparticles CuxMg1-xFe2O4 (x = 0, 0.5, 1) were synthesized by the co-precipitation method. The structure, morphological, optical, and magnetic properties of these spinel ferrites are investigated 2. EXPERIMENTAL SECTION Materials: Ferric chloride hexahydrate FeCl3⋅6H2O (98 %, Xilong-China), copper chloride hydrate CuCl2.2H2O (99%, Xilong-China), magnesium chloride hydrate MgCl2⋅6H2O (99 %, Xilong-China), sodium hydroxide NaOH (99 %, Xilong-China), ethanol (99 %, Xilong-China), deionized water (D.I). All chemicals were used as such without any purification. Characterization techniques: Structure and crystallinity were analyzed by Research Journal of Military Science and Technology, Special Issue, No.72A, 5 - 2021 47 X'Pert PRO PANalytical with a 0.15405 nm Cu-Kα radiation source. The morphology, particle size and elemental analysis of the samples were determined by a scanning electron microscope energy dispersive X-ray spectroscopy (SEM - EDX Hitachi S-4600). Fourier transform infrared spectroscopy (FTIR, TENSOR II, Bruker) was employed to investigate the surface functional group adsorbent of prepared nanostructured samples. Optical absorption spectra were measured by UV-vis spectrophotometer (Jasco V730). Magnetization measurements were carried out with a vibrating sample magnetometer (VSM) at room temperature. Synthesis of CuxMg1-xFe2O4 (x= 0, 0.5, 1) nanoparticles: The CuxMg1-xFe2O4 (x=0, 0.5, 1) nanoparticles were prepared using a chemical co-precipitation method [19]. The molar ratio of M 2+ /Fe 3+ (M = Cu, Mg) was kept constant at 1:2. These materials in the stoichiometric ratio were dissolved in 100 ml deionized water and was vigorously stirred using a magnetic agitator for 15 minutes. A solution NaOH 5M was dropped to adjust the pH = (9 - 10). The precipitate was heated at 90 o C under magnetic stirring for 2 hours. After cooling, the solid materials were filtered and washed several times by D.I and ethanol. The washed samples were dried at 100 o C for 12 hours and then calcined at temperatures 900 o C for 3 hours. 3. RESULTS AND DISCUSSION SEM analysis of the nanoparticles Figure 1. SEM images of (a) CuFe2O4, (b) MgFe2O4 and (c) Cu0.5Mg0.5Fe2O4 nanoparticles. The morphologies of CuFe2O4, MgFe2O4 and Cu0.5Mg0.5Fe2O4 nanoparticles were studied by a scanning electron microscope (SEM) as shown in figure 1. The average size of CuFe2O4 nanoparticles is less than 100 nm with particle Chemistry & Environment T. V. Chinh, , P. K. N. Ho, “Structure, morphological, co-precipitation method.” 48 aggregation into larger particles. The micrograph of MgFe2O4 has formed relative spherical particles with nano diameters in the range of 30 - 40 nm. And from fig 1c, the particles of Cu0.5Mg0.5Fe2O4 are relatively uniform in size with an average particle size of approximately 30 nm. The SEM observation of samples showed that the average size is in good agreement with their crystallite sizes determined from XRD spectra. XRD analysis of the samples XRD patterns of the CuFe2O4, MgFe2O4 and Cu0.5Mg0.5Fe2O4 nanoparticles were analyzed using XRD and shown in fig.2. As can be seen in fig.2, all the diffraction peaks at 19.05 o , 30.35 o , 35.08 o , 37.77 o , 43.97 o , 54.36 o , 58.4 o , 62.48 o , 65.09 o and 67.23 o , 74.25 o . The peaks correspond to the (111), (220), (311), (222), (400), (422), (511) (440 ... n of spinel CuFe2O4 (JCPDS 01-077-0010) and spinel MgFe2O4 (JCPDS 01-089-3084). Moreover, the formation of the secondary phase of Fe2O3 (JCPDS 01-085-0599) was observed in XRD patterns of CuFe2O4, MgFe2O4. The secondary phase was also reported for other ferrites in literatures [20, 21]. While, the XRD pattern of Cu0.5Mg0.5Fe2O4 sample has only a single spinel phase with diffraction planes (200), (311), (400), (422), (511) and (440). Finally, these diffraction peaks of the CuFe2O4, MgFe2O4 and Cu0.5Mg0.5Fe2O4 are indexed to the cubic crystal structure of the spinel phase with the Fd-3m space group. The crystallite size for the most intense peak (311) plane was calculated by using the Debye-Scherrer formula: Where k, λ, B and ϴ are Scherrer constant (0.89), the X-ray wavelength (0.15406 nm), the width of the peak at half maximum intensity (radian) and the angle of diffraction, respectively [16]. The lattice parameter was also calculated from XRD data for all the samples using the equation: Where (hkl) are Miller indices. Table 1. Crystallite size and lattice parameter for the samples. Samples Crystallite size (nm) Lattice parameter (Å ) CuFe2O4 (x = 1) 32.9 8.4836 MgFe2O4 (x = 0) 27.9 8.2785 Cu0.5Mg0.5Fe2O4 (x = 0.5) 29.5 8.2940 The crystallite size and the lattice parameter of the samples is shown in table 1. Research Journal of Military Science and Technology, Special Issue, No.72A, 5 - 2021 49 Figure 2. XRD patterns of the CuFe2O4, MgFe2O4 and Cu0.5Mg0.5Fe2O4. The calculated lattice parameter for MgFe2O4 is smaller than the lattice parameter for CuFe2O4. This can be explained on the basis of ionic radius, where the ionic radius of Mg 2+ ion (0.72 Å) [22] is smaller than that of Cu2+ ion (0.73 Å) [22]. When a larger Cu 2+ ion is replaced by a smaller Mg 2+ ion, the lattice parameter for Cu0.5Mg0.5Fe2O4 is smaller than the parameter for CuFe2O4 and larger than the parameter for MgFe2O4. EDX analysis of the nanoparticles Figure 3. EDX analysis of (a) CuFe2O4, (b) MgFe2O4 and (c) Cu0.5Mg0.5Fe2O4. Chemistry & Environment T. V. Chinh, , P. K. N. Ho, “Structure, morphological, co-precipitation method.” 50 Elemental analysis of CuFe2O4, MgFe2O4 and Cu0.5Mg0.5Fe2O4 samples based on EDX study (fig. 3). Fig. 3 shows the presence of peaks related to their constituent elements, with no other impurity. The Cu:Fe; Mg:Fe, and (Cu+Mg):Fe atomic ratios estimated by EDX analysis were (14.67:24.65), (13.69:25.52) and (12.8:23.17) which are nearly closeto the theoretical ratio of 1:2 in CuFe2O4, MgFe2O4 and Cu0.5Mg0.5Fe2O4, respectively. FT-IR and Magnetization analysis of the nanoparticles The Fourier transform infrared (FTIR) spectra of CuFe2O4, MgFe2O4 and Cu0.5Mg0.5Fe2O4 are presented in fig 4a. For three samples, the peaks around 3400 cm -1 and 1600 cm -1 are the contribution from the vibration of O-H group of adsorbed water or humidity on the surface [23, 24]. In the spinel ferrite structure, the metal ions are situated in two different sub-lattices designated as tetrahedral (A-site) and octahedral (B-site). The highest IR band ν1, is generally observed in the higher frequency range of 600 - 550 cm -1 , corresponding to the intrinsic stretching vibration of the metal-oxygen bond at the tetrahedral site Mtetra-O (A- site). The lowest IR band ν2, is usually observed in the frequency range of 450 - 385 cm -1 , assigned to stretching vibrations of the metal-oxygen bond at the octahedral site Mocta-O (B-site) [25, 26]. The values of ν1 and ν2 for samples are given in table 2 and confirm the formation of a spinel ferrite structure. Table 2. Absorption band (ν1 and ν2) of the samples. Samples ν1 (cm -1 ) ν2 (cm -1 ) CuFe2O4 (x = 1) 583.11 434.24 MgFe2O4 (x = 0) 576.13 436.95 Cu0.5Mg0.5Fe2O4 (x = 0.5) 576.95 433.98 Figure 4. (a) FTIR spectra and (b) Magnetic hysteresis loops of CuFe2O4, MgFe2O4, Cu0.5Mg0.5Fe2O4 nanoparticles. Hysteresis loops depicting the variation of magnetization (M, emu/g) and magnetic field (H, Oe) are shown in fig. 4b. The saturation magnetization (Ms), remanent magnetization (Mr) and coercivity (Hc) for all the samples are listed in the table 3. It was seen that the hysteresis loop of CuFe2O4 bears typical Research Journal of Military Science and Technology, Special Issue, No.72A, 5 - 2021 51 characteristics of ferromagnetic materials, while MgFe2O4 and Cu0.5Mg0.5Fe2O4 show superparamagnetic behavior. The values of Ms, Mr, Hc of CuFe2O4 are higher than that of both MgFe2O4 and Cu0.5Mg0.5Fe2O4. The saturation magnetization of MgFe2O4 is exceptionally low, after replacing Mg 2+ by Cu 2+ to enhance the Ms value of MgFe2O4. Table 3. Saturation magnetization (Ms), remanent magnetization (Mr) and coercivity (Hc) of the samples. Samples Ms (emu/g) Mr (emu/g) Hc (Oe) CuFe2O4 (x = 1) 29.5 13.6 560 MgFe2O4 (x = 0) 13.1 1.6 83 Cu0.5Mg0.5Fe2O4 (x = 0.5) 23.1 4.1 83 Ultraviolet - visible analysis of the samples Figure 5. (a) UV-vis absorption spectra and (b) Tauc plot of the CuFe2O4, MgFe2O4, Cu0.5Mg0.5Fe2O4 nanoparticles. The UV-vis absorption spectra of CuFe2O4, MgFe2O4 and Cu0.5Mg0.5Fe2O4 is shown in fig.6a. All the samples show a wide range of absorption from UV to visible region (200 nm - 850 nm). The optical absorption strength depends on the difference between the photon energy and the band gap as shown in the equation: (αhυ)1/n = A(hυ - Eg) where h is Planck’s constant (6,626.10-34 Js), υ is the light speed (3.108 ms-1), α is the adsorption coefficient, Eg is the band gap energy and A is the absorption. The catalyst is assumed to be the direct transition type (n=1/2) for the determination of the band gap energy of the photocatalysts. By using the Tauc method, a plot (αhυ)2 vs hυ was developed as shown in figure 5(b) and the calculated band gaps of energy are 1.41 eV, 2.04 eV, 1.65 eV for CuFe2O4, MgFe2O4, Cu0.5Mg0.5Fe2O4 respectively, indicating that the samples may have visible-light photoactivity. 4. CONCLUSIONS In summary, CuxMg1.xFe2O4 (x = 1, 0.5, 0) nanoparticles were successfully synthesized by co-precipitation. At calcined 900 o C for 3 hours, Cu0.5Mg0.5Fe2O4 Chemistry & Environment T. V. Chinh, , P. K. N. Ho, “Structure, morphological, co-precipitation method.” 52 had a single phase cubic spinel structure, while CuFe2O4, MgFe2O4 had formation of secondary phase of Fe2O3 in XRD patterns. The particles sizes of CuFe2O4, Cu0.5Mg0.5Fe2O4 and MgFe2O4 were 32.9 nm, 27.9 nm and 29.5 nm, respestively. Cu 2+ -doping improves the magnetic properties of magnesium ferrite, as the saturation magnetization (Ms) is increased from 13.1 emu/g to 23.1 emu/g. These spinel ferrites had small band gap energies of 1.41 eV, 2.04 eV, 1.65 eV for CuFe2O4, MgFe2O4, Cu0.5Mg0.5Fe2O4, respectively, indicating that they are used as adsorbents/photocatalysts for application in wastewater treatment. Acknowledgement: The authors are grateful to the colleagues of the Department of Inorganic Chemistry, Institute of Chemistry and Materials. REFERENCES [1]. Maensiri, S., et al., “A simple route to synthesize nickel ferrite (NiFe2O4) nanoparticles using egg white,” Scripta materialia, Vol. 56(9), p. 797-800, (2007). [2]. Reddy, A.E., et al., “Construction of novel nanocomposite ZnO@ CoFe2 O4 microspheres grown on nickel foam for high performance electrochemical supercapacitors,” Analytical Methods, Vol. 10(2), p. 223-229, (2018). [3]. Popov, A.M., et al., “Determination of lithium in lithium-ionic conductors by laser-enhanced ionization spectrometry with laser ablation,” Journal of Analytical Atomic Spectrometry, Vol. 29(1), p. 176-184, (2014). [4]. Céspedes, E., et al.,“Bacterially synthesized ferrite nanoparticles for magnetic hyperthermia applications,” Nanoscale, Vol. 6(21), p. 12958- 12970, (2014). [5]. Srinivasan, S.Y., et al., “Applications of cobalt ferrite nanoparticles in biomedical nanotechnology,” Nanomedicine, Vol. 13(10), p. 1221-1238, (2018). [6]. Yoon, T.J., et al., “Multifunctional nanoparticles possessing a “magnetic motor effect” for drug or gene delivery,” Angewandte Chemie, Vol. 117(7), p. 1092-1095, (2005). [7]. 7. Šutka, A. and K.A. Gross, “Spinel ferrite oxide semiconductor gas sensors,” Sensors Actuators B: Chemical, Vol. 222, p. 95-105, (2016). [8]. Salman, G., A. Bohan, and G. Jaed, “Use of Nano-Magnetic Material for Removal of Heavy Metals from Wastewater,” Engineering Technology Journal, Vol. 35(9 Part (A) Engineering), p. 903-908, (2017). [9]. Vamvakidis, K., et al., “Diverse Surface Chemistry of Cobalt Ferrite Nanoparticles to Optimize Copper (II) Removal from Aqueous Media,” Materials, Vol. 13(7), p. 1537, (2020). [10]. Abraham, A.G., et al., “Enhanced magneto-optical and photo-catalytic properties of transition metal cobalt (Co 2+ ions) doped spinel MgFe2O4 ferrite nanocomposites,” Journal of Magnetism Magnetic Materials, Vol. 452, p. 380-388, (2018). [11]. rti - ui one , J.-L., U. Pal, and M.S. Villanueva, “Structural, magnetic, and catalytic evaluation of spinel Co, Ni, and Co–Ni ferrite nanoparticles fabricated by low-temperature solution combustion process,” ACS omega, Vol. 3(11), p. 14986-15001, (2018). Research Journal of Military Science and Technology, Special Issue, No.72A, 5 - 2021 53 [12]. Lassoued, A., et al., “Synthesis and magnetic characterization of Spinel ferrites MFeO (M= Ni, Co, Zn and Cu) via chemical co-precipitation method,” Journal of Materials Science: Materials in Electronics, Vol. 28(24), (2017). [13]. Bhandare, S.V., et al., “Mechanistic insights into the sol-gel synthesis of complex (quaternary) Co–Mn–Zn-spinel ferrites: An annealing dependent study,” Ceramics International, Vol. 46(11), p. 17400-17415, (2020). [14]. Mohammad, A.M., S.M.A. Ridha, and T.H. Mubarak, “Dielectric properties of Cr-substituted cobalt ferrite nanoparticles synthesis by citrate-gel auto combustion method,” International Journal of Applied Engineering Research, Vol. 13(8), p. 6026-6035, (2018). [15]. Bid, S. and S. Pradhan, “Preparation of zinc ferrite by high-energy ball- milling and microstructure characterization by Rietveld’s analysis,” Materials Chemistry Physics, Vol. 82(1), p. 27-37, (2003). [16]. Nguyen, L.T., et al., “A Facile Synthesis, Characterization, and Photocatalytic Activity of Magnesium Ferrite Nanoparticles via the Solution Combustion Method,” Journal of Chemistry, Vol. 2019, (2019). [17]. Kader, S.S., D.P. Paul, and S.M. Hoque, “Effect of temperature on the structural and magnetic properties of CuFe2O4 nano particle prepared by chemical co-precipitation method,” International Journal of Materials, Mechanics Manufacturing, Vol. 2(1), p. 5-8, (2014). [18]. Anandan, S., et al., “Magnetic and catalytic properties of inverse spinel CuFe2O4 nanoparticles,” Journal of Magnetism and Magnetic Materials, Vol. 432, p. 437-443, (2017). [19]. Shih, Y.-J., et al., “Synthesis of magnetically recoverable ferrite (MFe2O4, M Co, Ni and Fe)-supported TiO2 photocatalysts for decolorization of methylene blue,” Catalysis Communications, Vol.72, p. 127-132, (2015). [20]. Zaki, H., S. Al-Heniti, and T. Elmosalami, “Structural, magnetic and dielectric studies of copper substituted nano-crystalline spinel magnesium zinc ferrite,” Journal of Alloys compounds, Vol. 633, p. 104-114, (2015). [21]. Airimioaei, M., et al., “Synthesis and functional properties of the Ni1−xMnxFe2O4 ferrites,” Journal of alloys compounds, Vol. 509(31), p. 8065- 8072, (2011). [22]. Shannon, R.D., “Revised effective ionic radii and systematic studies of interatomic distances in halides and chalcogenides,” Acta crystallographica section A: crystal physics, diffraction, theoretical general crystallography, Vol. 32(5), p. 751-767, (1976). [23]. Nguyen, T.B. and R.-a.J.R.A. Doong, “Heterostructured ZnFe2 O4/TiO2 nanocomposites with a highly recyclable visible-light-response for bisphenol A degradation,” RSC Adv, Vol. 7(79), p. 50006-50016 (2016). [24]. Manimozhi, V., et al., “Preparation and characterization of ferrite nanoparticles for the treatment of industrial waste water,” Digest Journal of Nanomaterials and Biostructures, Vol. 11(3), p. 1017-1027, (2016). [25]. Sezgin, N., et al., “Synthesis, characterization and, the heavy metal removal efficiency of MFe2O4 (M= Ni, Cu) nanoparticles,” Ekoloji, Vol. 22(89), p. 89-96, (2013). Chemistry & Environment T. V. Chinh, , P. K. N. Ho, “Structure, morphological, co-precipitation method.” 54 [26]. Raju, M.K.J.C.S.T., “FT-IR studies of Cu substituted Ni-Zn ferrites for structural and vibrational investigations,” Chemical Science Transactions, Vol. 4(1), p. 137-142, (2015). TÓM TẮT CẤU TRÚC, HÌNH THÁI HỌC, TỪ TÍNH VÀ TÍNH CHẤT QUANG CỦA NANO FERRITE CuxMg1-xFe2O4 (x = 0, 0.5, 1) TỔNG HỢP BẰNG PHƯƠNG PHÁP ĐỒNG KẾT TỦA Các hạt nano CuxMg1-xFe2O4 đã được tổng hợp thành công bằng phương pháp đồng kết tủa. Các mẫu được nung ở 900 oC trong 3 giờ, theo phổ XRD mẫu Cu0.5Mg0.5Fe2O4 có cấu trúc đơn pha dạng lập phương, trong khi đó, mẫu CuFe2O4 và MgFe2O4 có sự xuất hiện của pha Fe2O3. Từ độ bão hòa của Cu0.5Mg0.5Fe2O4 nằm trong khoảng giữa giá trị từ độ bão hòa của CuFe2O4 và MgFe2O4. CuFe2O4 là vật liệu sắt từ, Cu0.5Mg0.5Fe2O4 và MgFe2O4 là vật liệu siêu thuận từ. Tất cả các spinel ferrite được đánh giá một số tính chất như SEM, FT-IR, EDS và phổ UV-vis. Từ khóa: Spinel ferrite; Hạt nano; Vật liệu sắt từ; Siêu thuận từ. Received Jan 11 th 2021 Revised Jan 26 th 2021 Published May 10 th 2021 Author affiliations: 1 Institute of Chemistry and Materials; 2 Key Laboratory for Petrochemical and Refinery Technologies. *Corresponding author: chinhpkkq@gmail.com.
File đính kèm:
structure_morphological_magnetic_and_optical_properties_of_c.pdf