Electrochemical performance of hard carbon anode in different carbonate-Based electrolytes
The electrolytes of 1 M NaFSI dissolved in various carbonate solvents containing EC, PC and/or DMC have been
investigated to find out the most compatible electrolyte composition with hard carbon (HC) anode in Na-ion batteries
(NIBs). The physical properties including viscosity and conductivity were measured to correlate with the
electrochemical behaviors of these electrolytes. The Na/HC half-cell was used for testing the charge/discharge
performance of prepared electrolytes at room temperature. This best medium for the solvation of NaFSI salt is the
mixture of EC, PC and DMC with the ratio 3:1:1, respectively. Indeed, this electrolyte delivered a highest capacity of
335.6 mAh.g-1, excellent capacity retention of 73.7 % for 100 cycles.
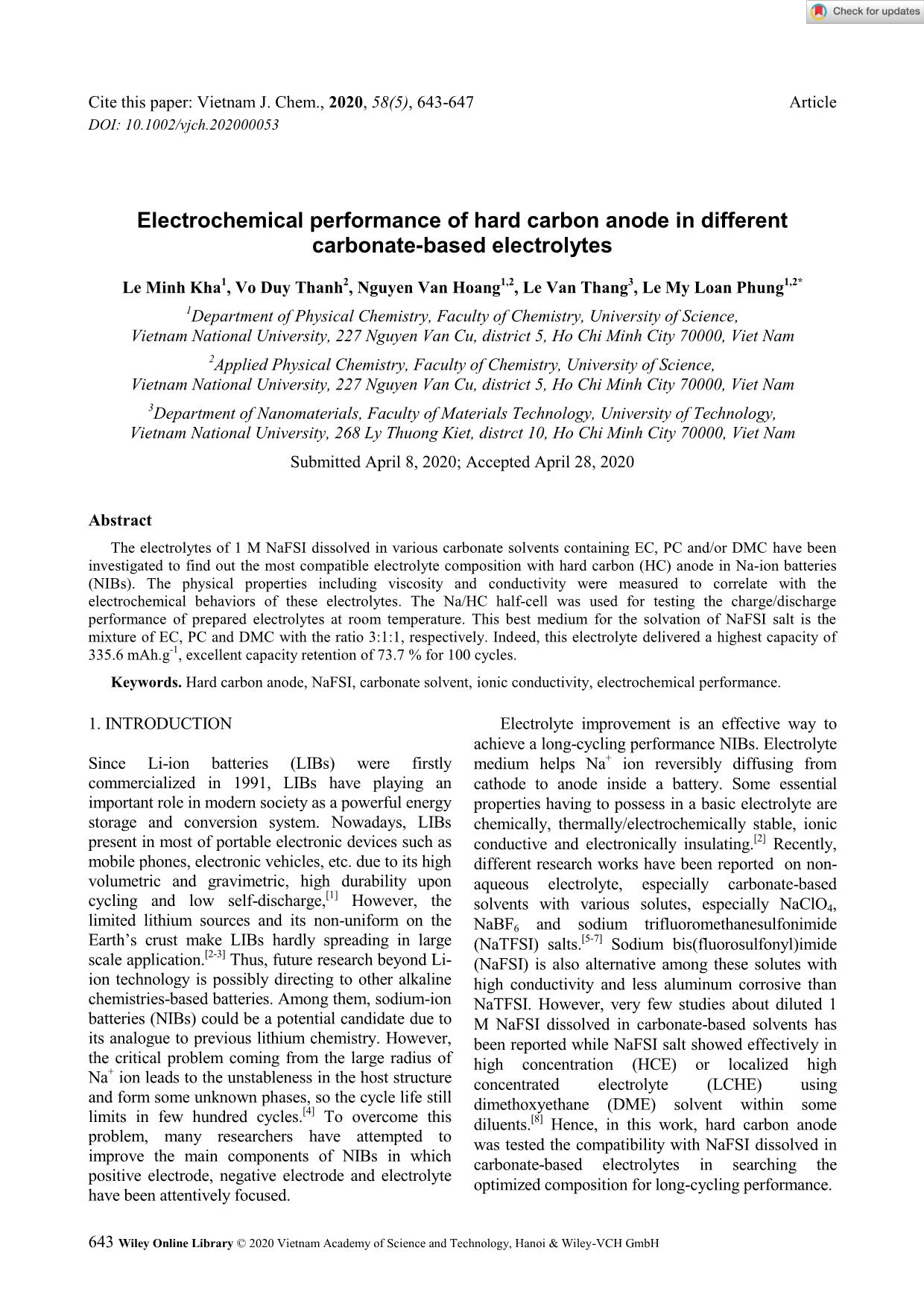
Trang 1
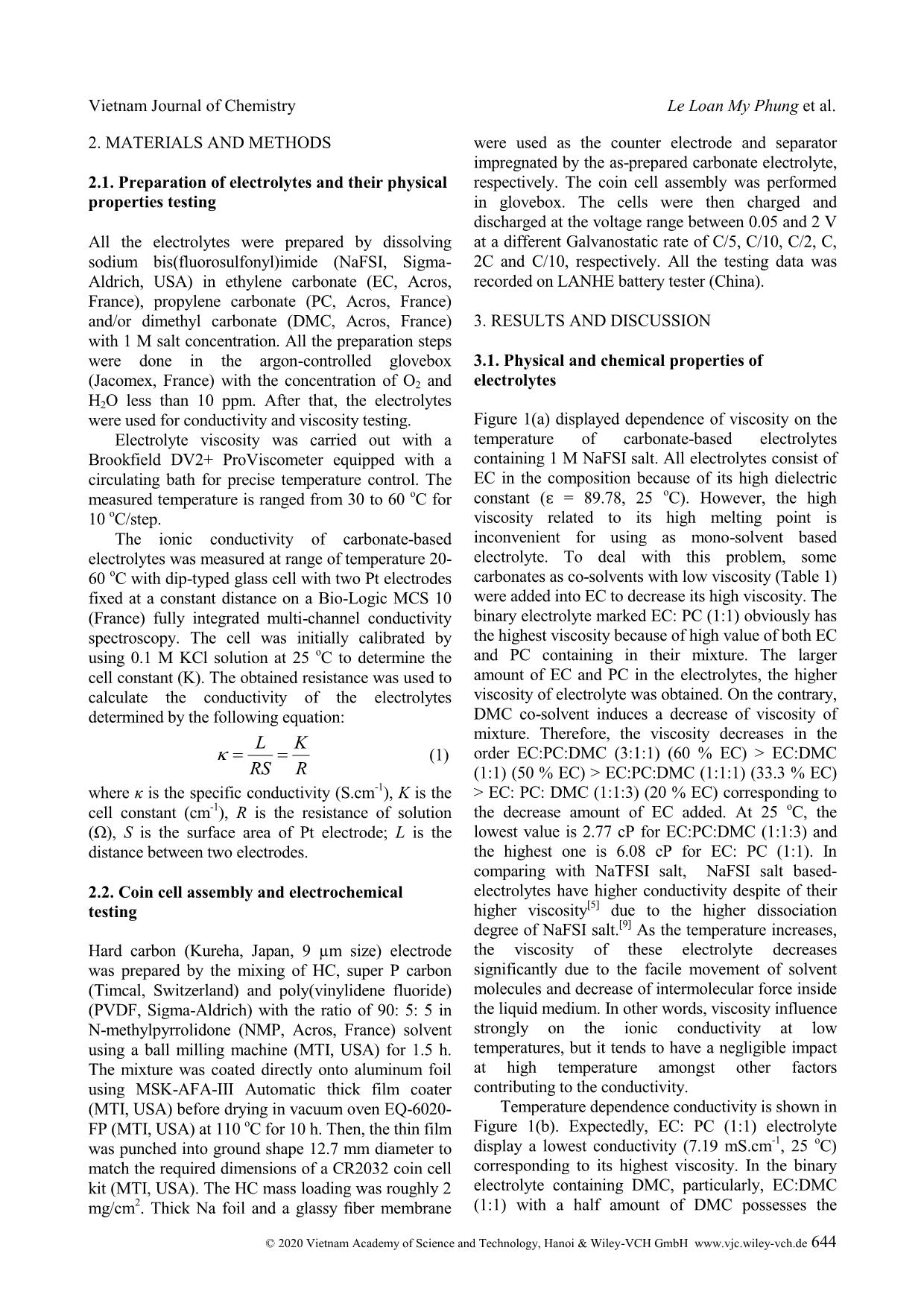
Trang 2
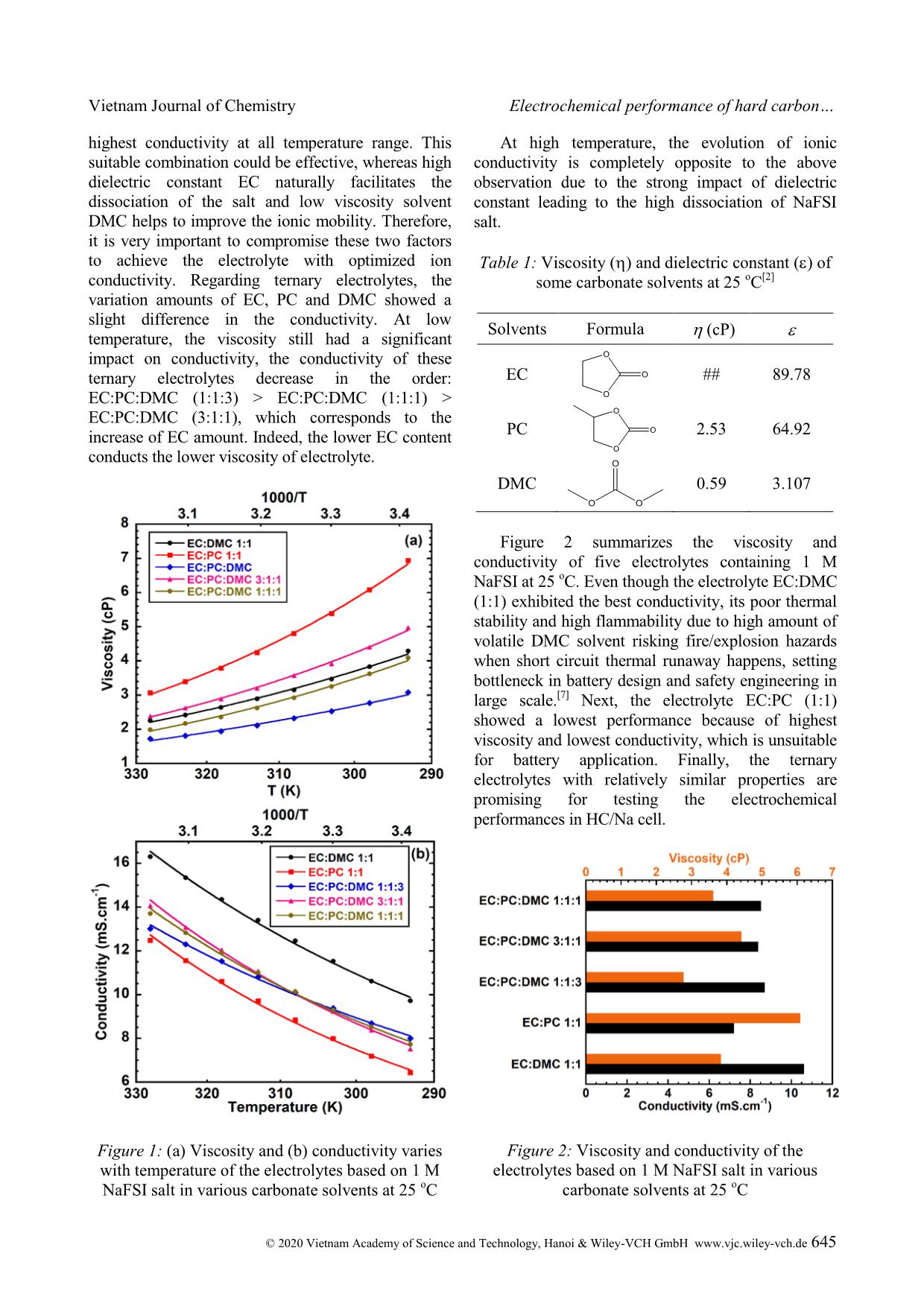
Trang 3
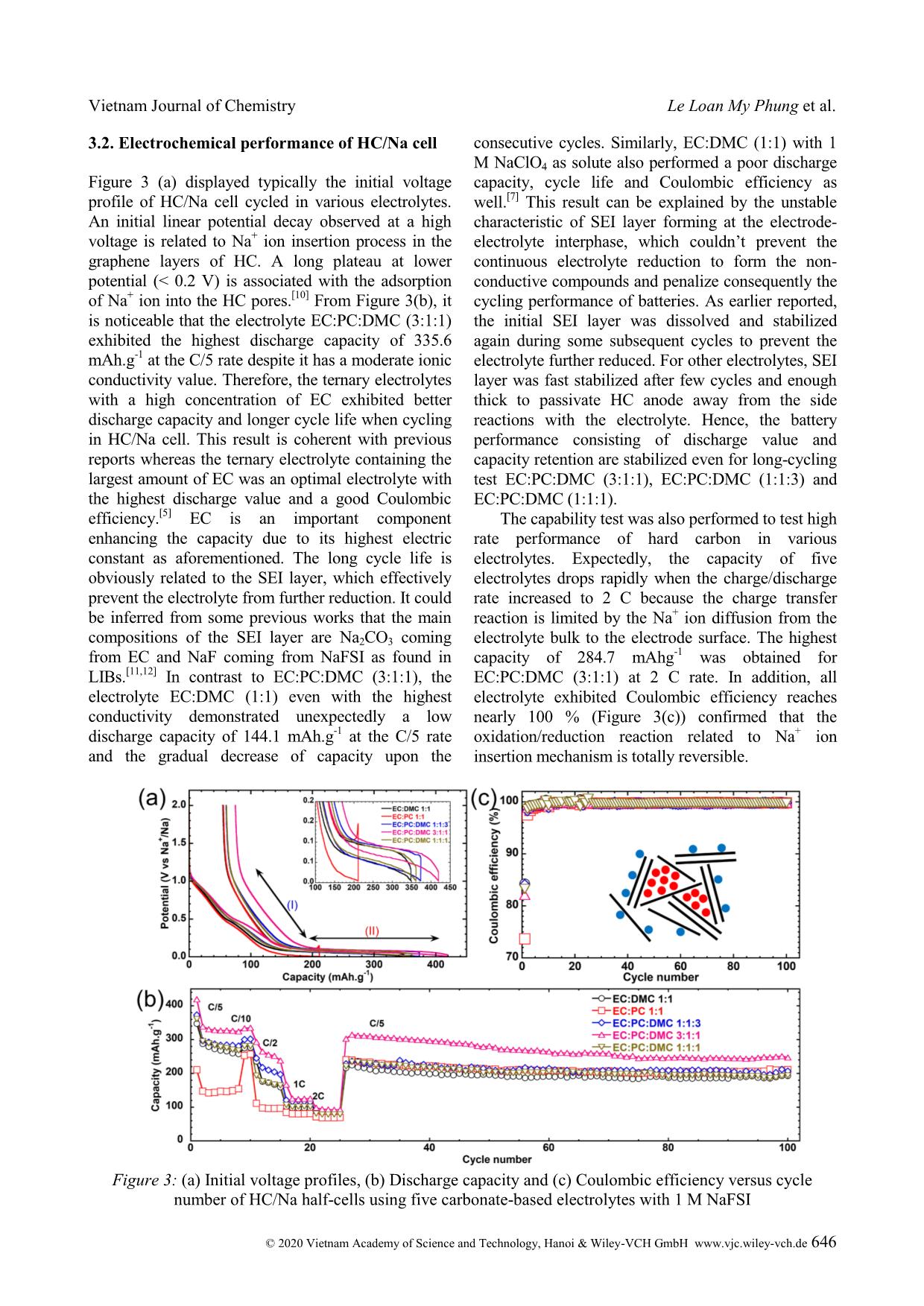
Trang 4
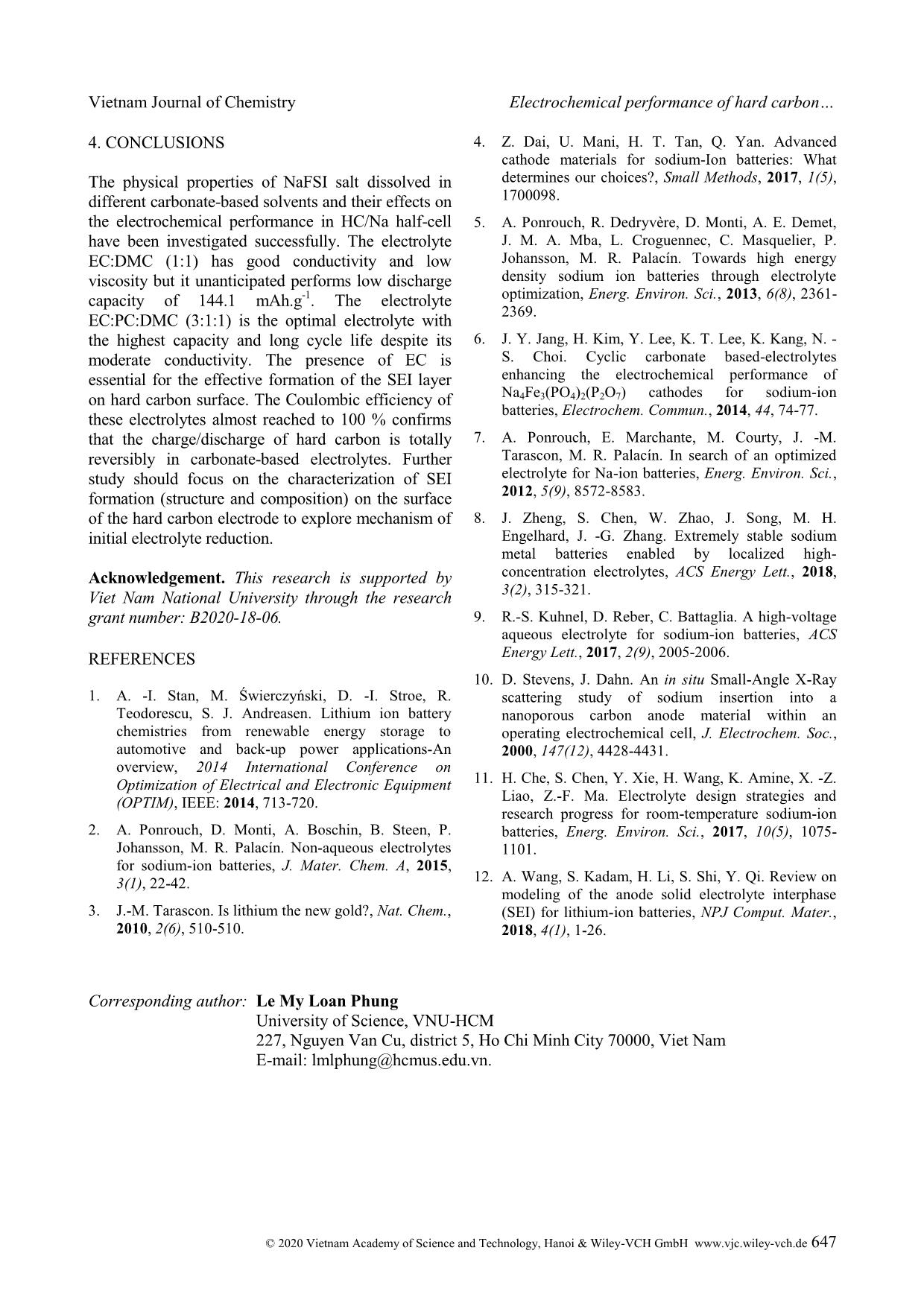
Trang 5
Tóm tắt nội dung tài liệu: Electrochemical performance of hard carbon anode in different carbonate-Based electrolytes
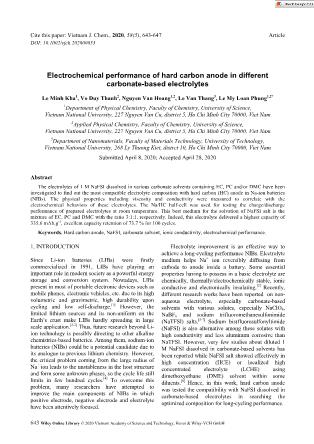
Cite this paper: Vietnam J. Chem., 2020, 58(5), 643-647 Article DOI: 10.1002/vjch.202000053 643 Wiley Online Library © 2020 Vietnam Academy of Science and Technology, Hanoi & Wiley-VCH GmbH Electrochemical performance of hard carbon anode in different carbonate-based electrolytes Le Minh Kha 1 , Vo Duy Thanh 2 , Nguyen Van Hoang 1,2 , Le Van Thang 3 , Le My Loan Phung 1,2* 1Department of Physical Chemistry, Faculty of Chemistry, University of Science, Vietnam National University, 227 Nguyen Van Cu, district 5, Ho Chi Minh City 70000, Viet Nam 2Applied Physical Chemistry, Faculty of Chemistry, University of Science, Vietnam National University, 227 Nguyen Van Cu, district 5, Ho Chi Minh City 70000, Viet Nam 3Department of Nanomaterials, Faculty of Materials Technology, University of Technology, Vietnam National University, 268 Ly Thuong Kiet, distrct 10, Ho Chi Minh City 70000, Viet Nam Submitted April 8, 2020; Accepted April 28, 2020 Abstract The electrolytes of 1 M NaFSI dissolved in various carbonate solvents containing EC, PC and/or DMC have been investigated to find out the most compatible electrolyte composition with hard carbon (HC) anode in Na-ion batteries (NIBs). The physical properties including viscosity and conductivity were measured to correlate with the electrochemical behaviors of these electrolytes. The Na/HC half-cell was used for testing the charge/discharge performance of prepared electrolytes at room temperature. This best medium for the solvation of NaFSI salt is the mixture of EC, PC and DMC with the ratio 3:1:1, respectively. Indeed, this electrolyte delivered a highest capacity of 335.6 mAh.g -1 , excellent capacity retention of 73.7 % for 100 cycles. Keywords. Hard carbon anode, NaFSI, carbonate solvent, ionic conductivity, electrochemical performance. 1. INTRODUCTION Since Li-ion batteries (LIBs) were firstly commercialized in 1991, LIBs have playing an important role in modern society as a powerful energy storage and conversion system. Nowadays, LIBs present in most of portable electronic devices such as mobile phones, electronic vehicles, etc. due to its high volumetric and gravimetric, high durability upon cycling and low self-discharge, [1] However, the limited lithium sources and its non-uniform on the Earth’s crust make LIBs hardly spreading in large scale application. [2-3] Thus, future research beyond Li- ion technology is possibly directing to other alkaline chemistries-based batteries. Among them, sodium-ion batteries (NIBs) could be a potential candidate due to its analogue to previous lithium chemistry. However, the critical problem coming from the large radius of Na + ion leads to the unstableness in the host structure and form some unknown phases, so the cycle life still limits in few hundred cycles. [4] To overcome this problem, many researchers have attempted to improve the main components of NIBs in which positive electrode, negative electrode and electrolyte have been attentively focused. Electrolyte improvement is an effective way to achieve a long-cycling performance NIBs. Electrolyte medium helps Na + ion reversibly diffusing from cathode to anode inside a battery. Some essential properties having to possess in a basic electrolyte are chemically, thermally/electrochemically stable, ionic conductive and electronically insulating. [2] Recently, different research works have been reported on non- aqueous electrolyte, especially carbonate-based solvents with various solutes, especially NaClO4, NaBF6 and sodium trifluoromethanesulfonimide (NaTFSI) salts. [5-7] Sodium bis(fluorosulfonyl)imide (NaFSI) is also alternative among these solutes with high conductivity and less aluminum corrosive than NaTFSI. However, very few studies about diluted 1 M NaFSI dissolved in carbonate-based solvents has been reported while NaFSI salt showed effectively in high concentration (HCE) or localized high concentrated electrolyte (LCHE) using dimethoxyethane (DME) solvent within some diluents. [8] Hence, in this work, hard carbon anode was tested the compatibility with NaFSI dissolved in carbonate-based electrolytes in searching the optimized composition for long-cycling performance. Vietnam Journal of Chemistry Le Loan My Phung et al. © 2020 Vietnam Academy of Science and Technology, Hanoi & Wiley-VCH GmbH www.vjc.wiley-vch.de 644 2. MATERIALS AND METHODS 2.1. Preparation of electrolytes and their physical properties testing All the electrolytes were prepared by dissolving sodium bis(fluorosulfonyl)imide (NaFSI, Sigma- Aldrich, USA) in ethylene carbonate (EC, Acros, France), propylene carbonate (PC, Acros, France) and/or dimethyl carbonate (DMC, Acros, France) with 1 M salt concentration. All the preparation steps were done in the argon-controlled glovebox (Jacomex, France) with the concentration of O2 and H2O less than 10 ppm. After that, the electrolytes were used for conductivity and viscosity testing. Electrolyte viscosity was carried out with a Brookfield DV2+ ProViscometer equipped with a circulating bath for precise temperature control. The measured temperature is ranged from 30 to 60 o C for 10 o C/step. The ionic conductivity of carbonate-based electrolytes was measured at range of temperature 20- 60 o C with dip-typed glass cell with two Pt electrodes fixed at a constant distance on a Bio-Logic MCS 10 (France) fully integrated multi-channel conductivity spectroscopy. The cell was initially calibrated by using 0.1 M KCl solution at 25 o C to determine the cell constant (K). The obtained resistance was used to calculate the conductivity of the electrolytes determined by the following equation: L K RS R (1) where κ is the specific conductivity (S.cm-1), K is the cell constant (cm -1 ), R is the resistance of solution (Ω), S is the surface area of Pt electrode; L is the distance between two electrodes. 2.2. Coin cell assembly and electrochemical testing Hard carbon (Kureha, Japan, 9 µm size) electrode was prepared by the mixing of HC, super P carbon (Timcal, Switzerland) and poly(vinylidene fluoride) (PVDF, Sigma-Aldrich) with the ratio of 90: 5: 5 in N-methylpyrrolidone (NMP, Acros, France) solvent using a ball milling machine (MTI, USA) for 1.5 h. The mixture was coated directly onto aluminum foil using MSK-AFA-III Automatic thick film coater (MTI, USA) before drying in vacuum oven EQ-6020- FP (MTI, USA) at 110 o C for 10 h. Then, the thin film was punched into ground shape 12.7 mm diameter to match the required dimensions of a CR2032 coin cell kit (MTI, USA). The HC mass loading was roughly 2 mg/cm 2 . Thick Na foil and a glassy fiber membrane were used as the counter electrode and separator impregnated by the as-prepared carbonate electrolyte, respectively. The coin cell assembly was performed in glovebox. The cells were then charged and discharged at the voltage range between 0.05 and 2 V at a different Galvanostatic rate of C/5, C/10, C/2, C, 2C and C/10, respectively. All the testing data was recorded on LANHE battery tester (China). 3. RESULTS AND DISCUSSION 3.1. Physical and chemical properties of electrolytes Figure 1(a) displayed dependence of viscosity on the temperature of carbonate-based electrolytes containing 1 M NaFSI salt. All electrolytes consist of EC in the composition because of its high dielectric constant (ɛ = 89.78, 25 oC). However, the high viscosity related to its high melting point is inconvenient for using as mono-solvent based electrolyte. To deal with this problem, some carbonates as co-solvents with low viscosity (Table 1) were added into EC to decrease its high viscosity. The binary electrolyte marked EC: PC (1:1) obviously has the highest viscosity because of high value of both EC and PC containing in their mixture. The larger amount of EC and PC in the electrolytes, the higher viscosity of electrolyte was obtained. On the contrary, DMC co-solvent induces a decrease of viscosity of mixture. Therefore, the viscosity decreases in the order EC:PC:DMC (3:1:1) (60 % EC) > EC:DMC (1:1) (50 % EC) > EC:PC:DMC (1:1:1) (33.3 % EC) > EC: PC: DMC (1:1:3) (20 % EC) corresponding to the decrease amount of EC added. At 25 o C, the lowest value is 2.77 cP for EC:PC:DMC (1:1:3) and the highest one is 6.08 cP for EC: PC (1:1). In comparing with NaTFSI salt, NaFSI salt based- electrolytes have higher conductivity despite of their higher viscosity [5] due to the higher dissociation degree of NaFSI salt. [9] As the temperature increases, the viscosity of these electrolyte decreases significantly due to the facile movement of solvent molecules and decrease of intermolecular force inside the liquid medium. In other words, viscosity influence strongly on the ionic conductivity at low temperatures, but it tends to have a negligible impact at high temperature amongst other factors contributing to the conductivity. Temperature dependence conductivity is shown in Figure 1(b). Expectedly, EC: PC (1:1) electrolyte display a lowest conductivity (7.19 mS.cm -1 , 25 o C) corresponding to its highest viscosity. In the binary electrolyte containing DMC, particularly, EC:DMC (1:1) with a half amount of DMC possesses the Vietnam Journal of Chemistry Electrochemical performance of hard carbon © 2020 Vietnam Academy of Science and Technology, Hanoi & Wiley-VCH GmbH www.vjc.wiley-vch.de 645 highest conductivity at all temperature range. This suitable combination could be effective, whereas high dielectric constant EC naturally facilitates the dissociation of the salt and low viscosity solvent DMC helps to improve the ionic mobility. Therefore, it is very important to compromise these two factors to achieve the electrolyte with optimized ion conductivity. Regarding ternary electrolytes, the variation amounts of EC, PC and DMC showed a slight difference in the conductivity. At low temperature, the viscosity still had a significant impact on conductivity, the conductivity of these ternary electrolytes decrease in the order: EC:PC:DMC (1:1:3) > EC:PC:DMC (1:1:1) > EC:PC:DMC (3:1:1), which corresponds to the increase of EC amount. Indeed, the lower EC content conducts the lower viscosity of electrolyte. Figure 1: (a) Viscosity and (b) conductivity varies with temperature of the electrolytes based on 1 M NaFSI salt in various carbonate solvents at 25 o C At high temperature, the evolution of ionic conductivity is completely opposite to the above observation due to the strong impact of dielectric constant leading to the high dissociation of NaFSI salt. Table 1: Viscosity () and dielectric constant () of some carbonate solvents at 25 o C [2] Solvents Formula (cP) EC O O O ## 89.78 PC O O O 2.53 64.92 DMC O O O 0.59 3.107 Figure 2 summarizes the viscosity and conductivity of five electrolytes containing 1 M NaFSI at 25 o C. Even though the electrolyte EC:DMC (1:1) exhibited the best conductivity, its poor thermal stability and high flammability due to high amount of volatile DMC solvent risking fire/explosion hazards when short circuit thermal runaway happens, setting bottleneck in battery design and safety engineering in large scale. [7] Next, the electrolyte EC:PC (1:1) showed a lowest performance because of highest viscosity and lowest conductivity, which is unsuitable for battery application. Finally, the ternary electrolytes with relatively similar properties are promising for testing the electrochemical performances in HC/Na cell. Figure 2: Viscosity and conductivity of the electrolytes based on 1 M NaFSI salt in various carbonate solvents at 25 o C Vietnam Journal of Chemistry Le Loan My Phung et al. © 2020 Vietnam Academy of Science and Technology, Hanoi & Wiley-VCH GmbH www.vjc.wiley-vch.de 646 3.2. Electrochemical performance of HC/Na cell Figure 3 (a) displayed typically the initial voltage profile of HC/Na cell cycled in various electrolytes. An initial linear potential decay observed at a high voltage is related to Na + ion insertion process in the graphene layers of HC. A long plateau at lower potential (< 0.2 V) is associated with the adsorption of Na + ion into the HC pores. [10] From Figure 3(b), it is noticeable that the electrolyte EC:PC:DMC (3:1:1) exhibited the highest discharge capacity of 335.6 mAh.g -1 at the C/5 rate despite it has a moderate ionic conductivity value. Therefore, the ternary electrolytes with a high concentration of EC exhibited better discharge capacity and longer cycle life when cycling in HC/Na cell. This result is coherent with previous reports whereas the ternary electrolyte containing the largest amount of EC was an optimal electrolyte with the highest discharge value and a good Coulombic efficiency. [5] EC is an important component enhancing the capacity due to its highest electric constant as aforementioned. The long cycle life is obviously related to the SEI layer, which effectively prevent the electrolyte from further reduction. It could be inferred from some previous works that the main compositions of the SEI layer are Na2CO3 coming from EC and NaF coming from NaFSI as found in LIBs. [11,12] In contrast to EC:PC:DMC (3:1:1), the electrolyte EC:DMC (1:1) even with the highest conductivity demonstrated unexpectedly a low discharge capacity of 144.1 mAh.g -1 at the C/5 rate and the gradual decrease of capacity upon the consecutive cycles. Similarly, EC:DMC (1:1) with 1 M NaClO4 as solute also performed a poor discharge capacity, cycle life and Coulombic efficiency as well. [7] This result can be explained by the unstable characteristic of SEI layer forming at the electrode- electrolyte interphase, which couldn’t prevent the continuous electrolyte reduction to form the non- conductive compounds and penalize consequently the cycling performance of batteries. As earlier reported, the initial SEI layer was dissolved and stabilized again during some subsequent cycles to prevent the electrolyte further reduced. For other electrolytes, SEI layer was fast stabilized after few cycles and enough thick to passivate HC anode away from the side reactions with the electrolyte. Hence, the battery performance consisting of discharge value and capacity retention are stabilized even for long-cycling test EC:PC:DMC (3:1:1), EC:PC:DMC (1:1:3) and EC:PC:DMC (1:1:1). The capability test was also performed to test high rate performance of hard carbon in various electrolytes. Expectedly, the capacity of five electrolytes drops rapidly when the charge/discharge rate increased to 2 C because the charge transfer reaction is limited by the Na + ion diffusion from the electrolyte bulk to the electrode surface. The highest capacity of 284.7 mAhg -1 was obtained for EC:PC:DMC (3:1:1) at 2 C rate. In addition, all electrolyte exhibited Coulombic efficiency reaches nearly 100 % (Figure 3(c)) confirmed that the oxidation/reduction reaction related to Na + ion insertion mechanism is totally reversible. Figure 3: (a) Initial voltage profiles, (b) Discharge capacity and (c) Coulombic efficiency versus cycle number of HC/Na half-cells using five carbonate-based electrolytes with 1 M NaFSI Vietnam Journal of Chemistry Electrochemical performance of hard carbon © 2020 Vietnam Academy of Science and Technology, Hanoi & Wiley-VCH GmbH www.vjc.wiley-vch.de 647 4. CONCLUSIONS The physical properties of NaFSI salt dissolved in different carbonate-based solvents and their effects on the electrochemical performance in HC/Na half-cell have been investigated successfully. The electrolyte EC:DMC (1:1) has good conductivity and low viscosity but it unanticipated performs low discharge capacity of 144.1 mAh.g -1 . The electrolyte EC:PC:DMC (3:1:1) is the optimal electrolyte with the highest capacity and long cycle life despite its moderate conductivity. The presence of EC is essential for the effective formation of the SEI layer on hard carbon surface. The Coulombic efficiency of these electrolytes almost reached to 100 % confirms that the charge/discharge of hard carbon is totally reversibly in carbonate-based electrolytes. Further study should focus on the characterization of SEI formation (structure and composition) on the surface of the hard carbon electrode to explore mechanism of initial electrolyte reduction. Acknowledgement. This research is supported by Viet Nam National University through the research grant number: B2020-18-06. REFERENCES 1. A. -I. Stan, M. Świerczyński, D. -I. Stroe, R. Teodorescu, S. J. Andreasen. Lithium ion battery chemistries from renewable energy storage to automotive and back-up power applications-An overview, 2014 International Conference on Optimization of Electrical and Electronic Equipment (OPTIM), IEEE: 2014, 713-720. 2. A. Ponrouch, D. Monti, A. Boschin, B. Steen, P. Johansson, M. R. Palacín. Non-aqueous electrolytes for sodium-ion batteries, J. Mater. Chem. A, 2015, 3(1), 22-42. 3. J.-M. Tarascon. Is lithium the new gold?, Nat. Chem., 2010, 2(6), 510-510. 4. Z. Dai, U. Mani, H. T. Tan, Q. Yan. Advanced cathode materials for sodium-Ion batteries: What determines our choices?, Small Methods, 2017, 1(5), 1700098. 5. A. Ponrouch, R. Dedryvère, D. Monti, A. E. Demet, J. M. A. Mba, L. Croguennec, C. Masquelier, P. Johansson, M. R. Palacín. Towards high energy density sodium ion batteries through electrolyte optimization, Energ. Environ. Sci., 2013, 6(8), 2361- 2369. 6. J. Y. Jang, H. Kim, Y. Lee, K. T. Lee, K. Kang, N. - S. Choi. Cyclic carbonate based-electrolytes enhancing the electrochemical performance of Na4Fe3(PO4)2(P2O7) cathodes for sodium-ion batteries, Electrochem. Commun., 2014, 44, 74-77. 7. A. Ponrouch, E. Marchante, M. Courty, J. -M. Tarascon, M. R. Palacín. In search of an optimized electrolyte for Na-ion batteries, Energ. Environ. Sci., 2012, 5(9), 8572-8583. 8. J. Zheng, S. Chen, W. Zhao, J. Song, M. H. Engelhard, J. -G. Zhang. Extremely stable sodium metal batteries enabled by localized high- concentration electrolytes, ACS Energy Lett., 2018, 3(2), 315-321. 9. R.-S. Kuhnel, D. Reber, C. Battaglia. A high-voltage aqueous electrolyte for sodium-ion batteries, ACS Energy Lett., 2017, 2(9), 2005-2006. 10. D. Stevens, J. Dahn. An in situ Small-Angle X-Ray scattering study of sodium insertion into a nanoporous carbon anode material within an operating electrochemical cell, J. Electrochem. Soc., 2000, 147(12), 4428-4431. 11. H. Che, S. Chen, Y. Xie, H. Wang, K. Amine, X. -Z. Liao, Z.-F. Ma. Electrolyte design strategies and research progress for room-temperature sodium-ion batteries, Energ. Environ. Sci., 2017, 10(5), 1075- 1101. 12. A. Wang, S. Kadam, H. Li, S. Shi, Y. Qi. Review on modeling of the anode solid electrolyte interphase (SEI) for lithium-ion batteries, NPJ Comput. Mater., 2018, 4(1), 1-26. Corresponding author: Le My Loan Phung University of Science, VNU-HCM 227, Nguyen Van Cu, district 5, Ho Chi Minh City 70000, Viet Nam E-mail: lmlphung@hcmus.edu.vn.
File đính kèm:
electrochemical_performance_of_hard_carbon_anode_in_differen.pdf