Acetylcholinesterase sensor based on PANi/rGO film electrochemically grown on screen-Printed electrodes
In this work, the polyaniline/reduced graphene oxide (PANi/rGO) bilayer was directly electrodeposited on carbon
screen-printed electrodes (SPE). Some details in growth of PANi/rGO bilayer were revealed from cyclic
voltammograms and X-ray photoelectron spectra. The growth of stacked rGO film at high compactness on the electrode
surface is mainly accompanied with reduction of epoxy functional groups at basal planes of graphitic flakes. The asgrown rGO layer with abundent hydroxyl functional groups at basal planes is preferable to attract intrinsic fibrillar-like
PANi polymer chains in protonated aqueous media. The as-prepared PANi/rGO hybrid bilayer has shown good
conductivity, high porosity, good adhesion to biomolecules, and fast electron transfer rate (increased by 3.8 times).
Herein, PANi/rGO film has been further utilized to develop disposable acetylcholinesterase sensors able to detect
acetylthiocholine (ATCh) with apparent Michaelis - Menten constant of 0.728 mM. These sensors provide a very
promising technical solution for in-situ monitoring acetylthiocholine level in patients with neuro-diseases and
determination of neuro-toxins such as sarin and pesticides.
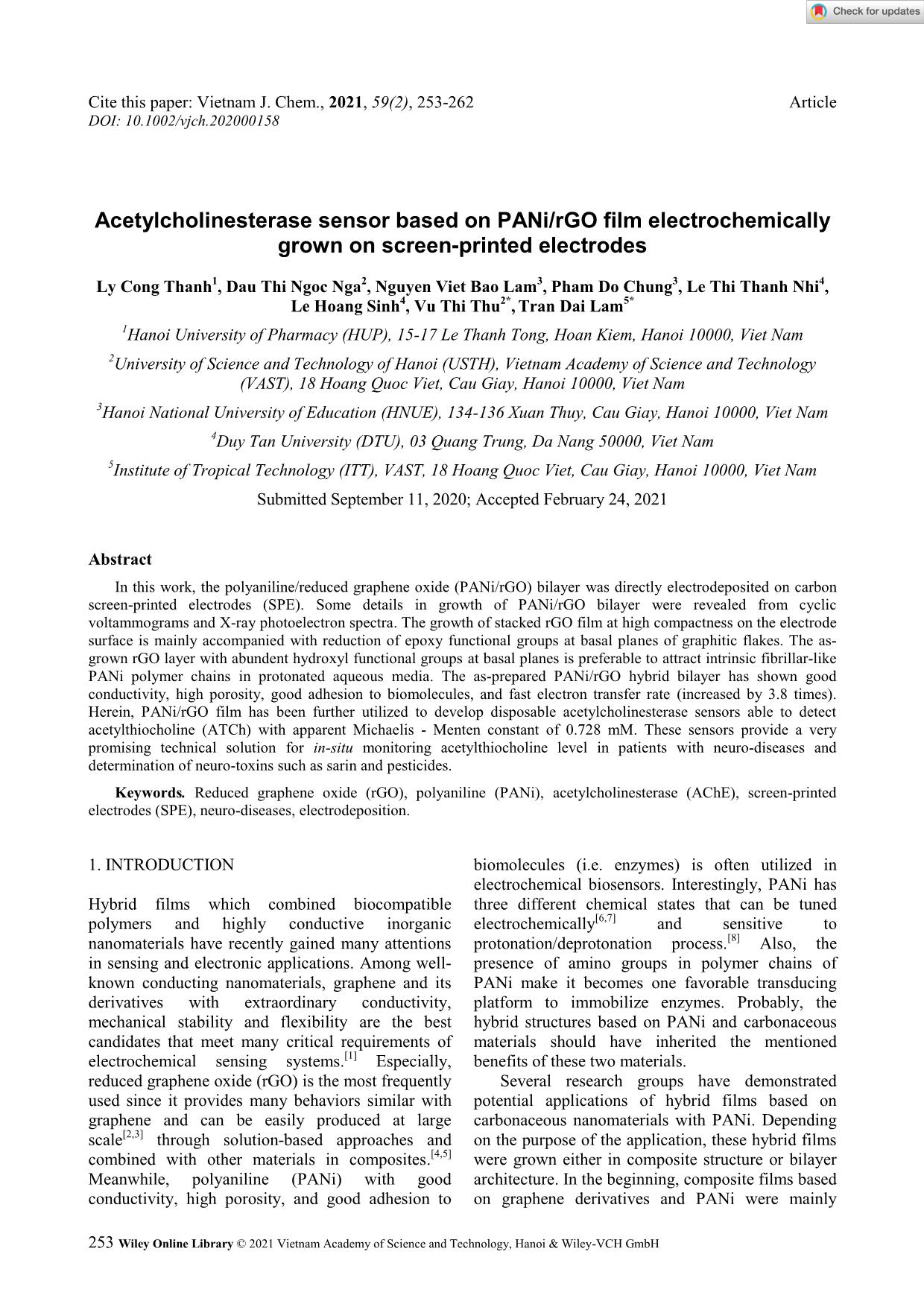
Trang 1
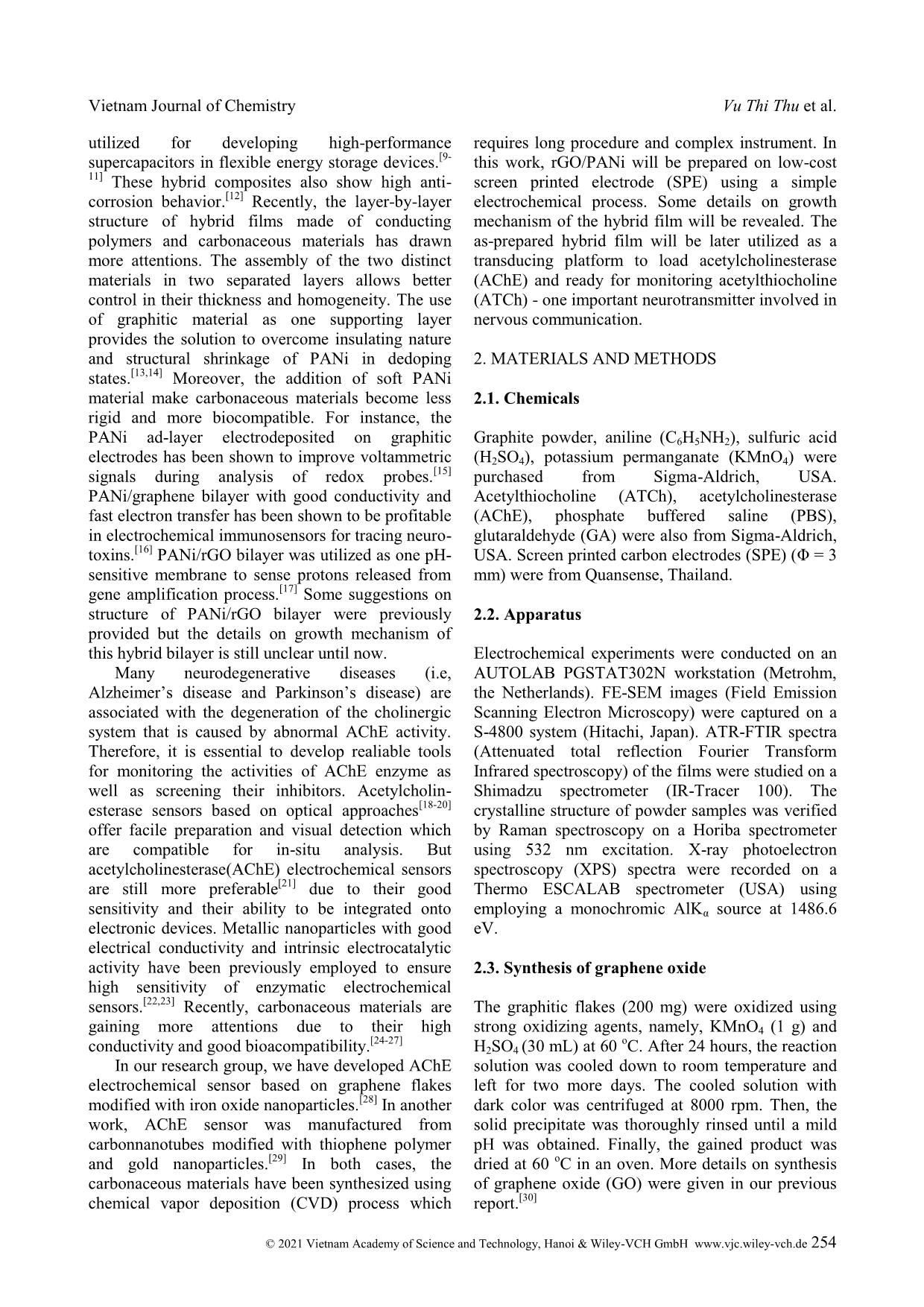
Trang 2
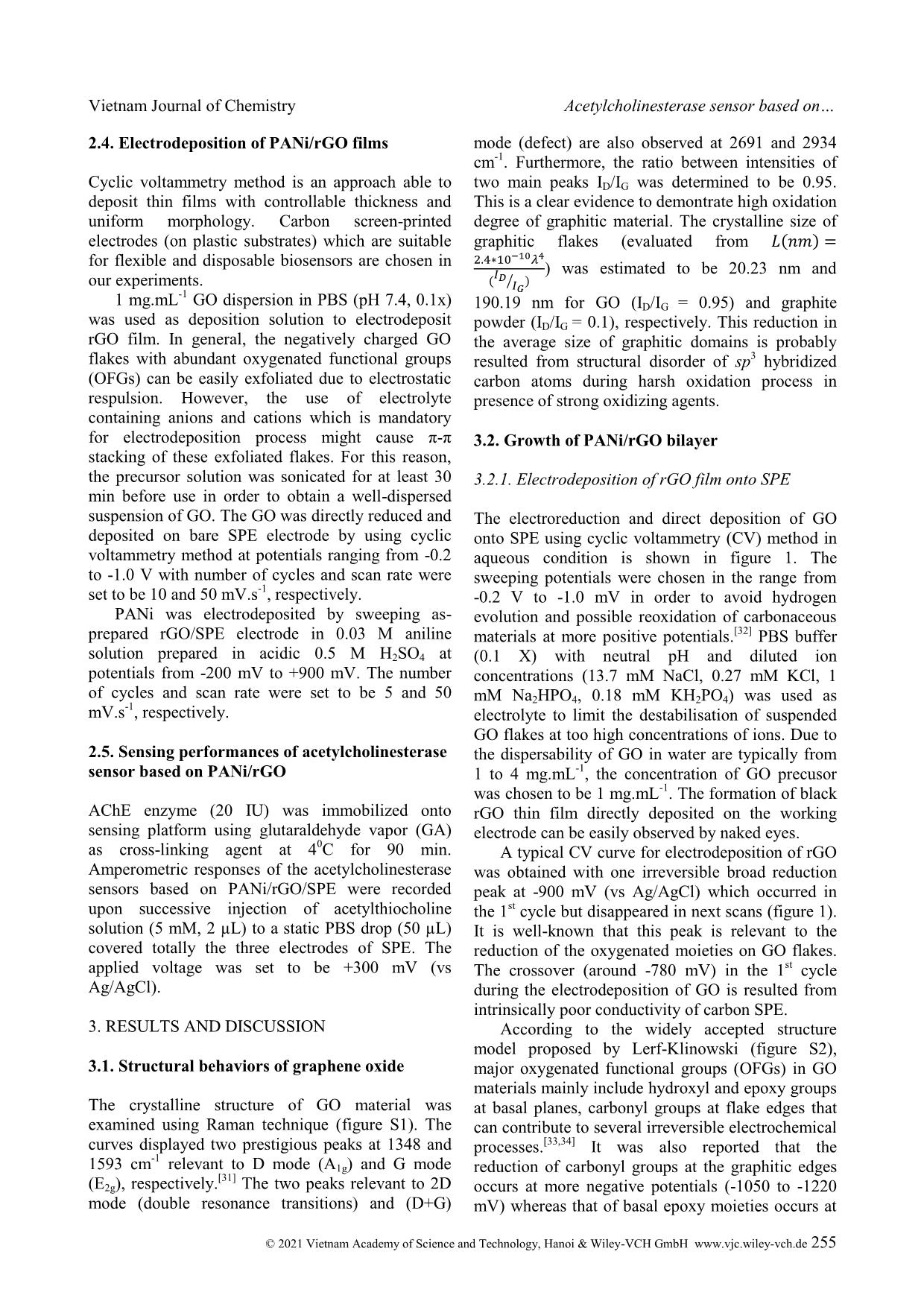
Trang 3
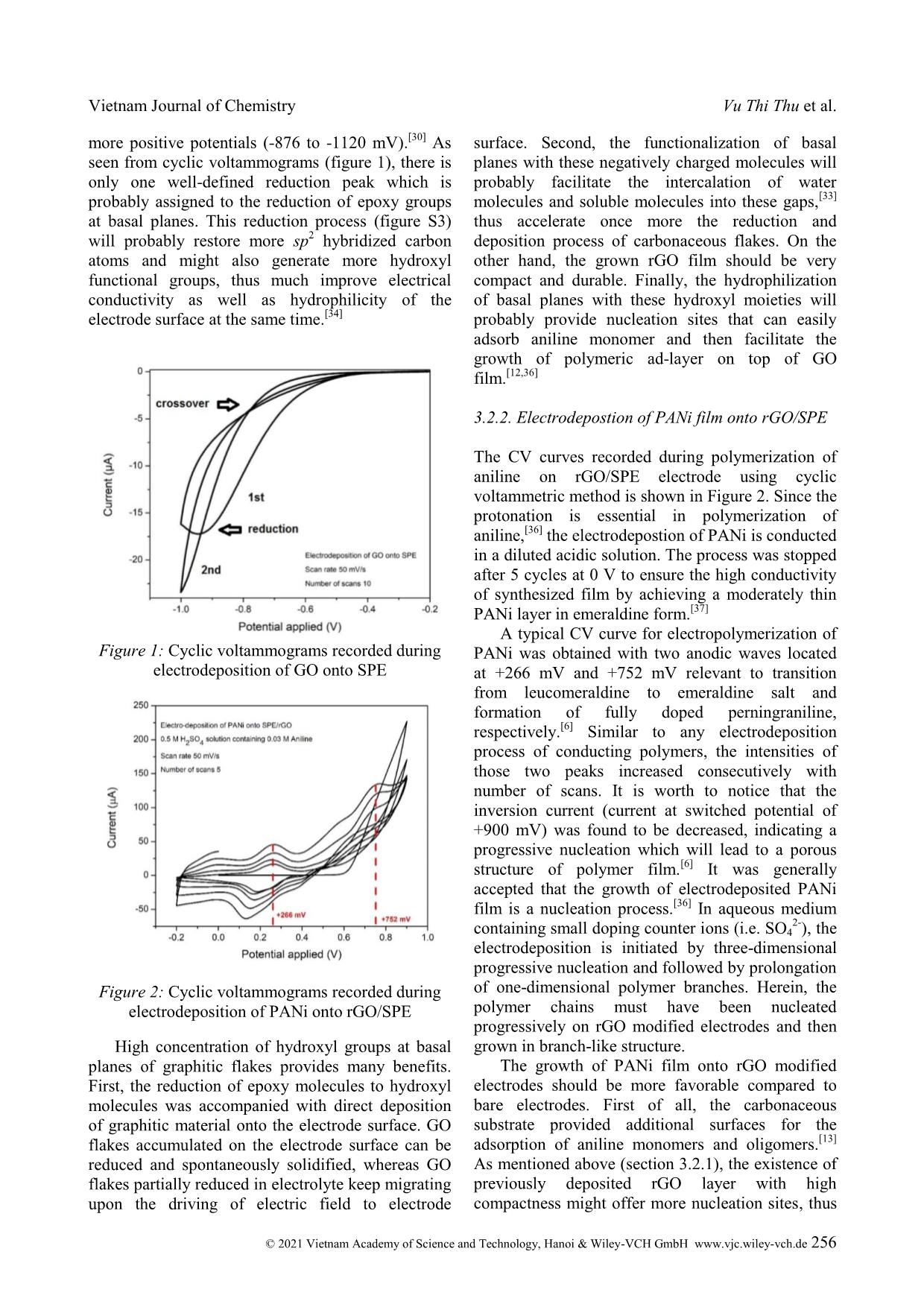
Trang 4
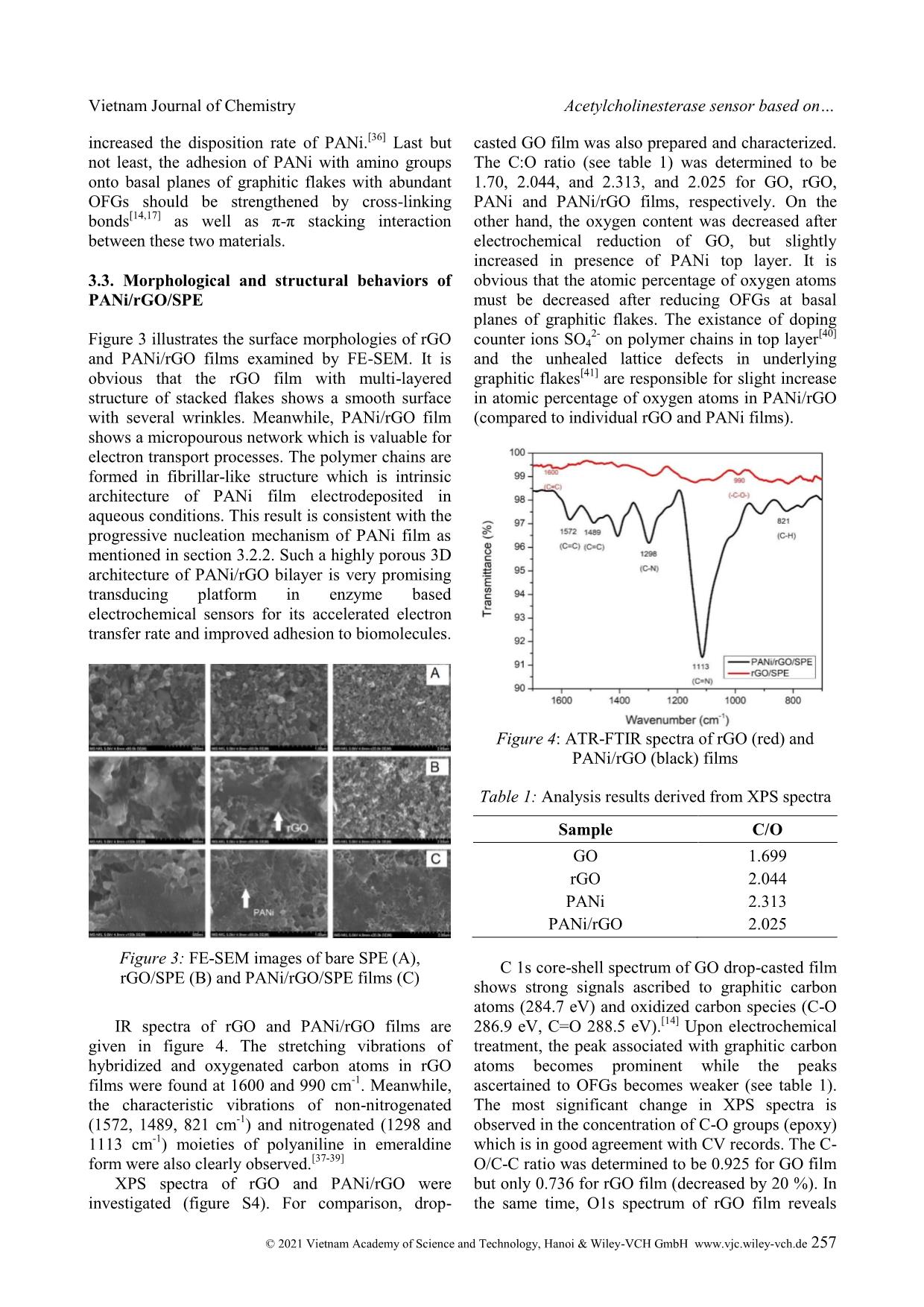
Trang 5
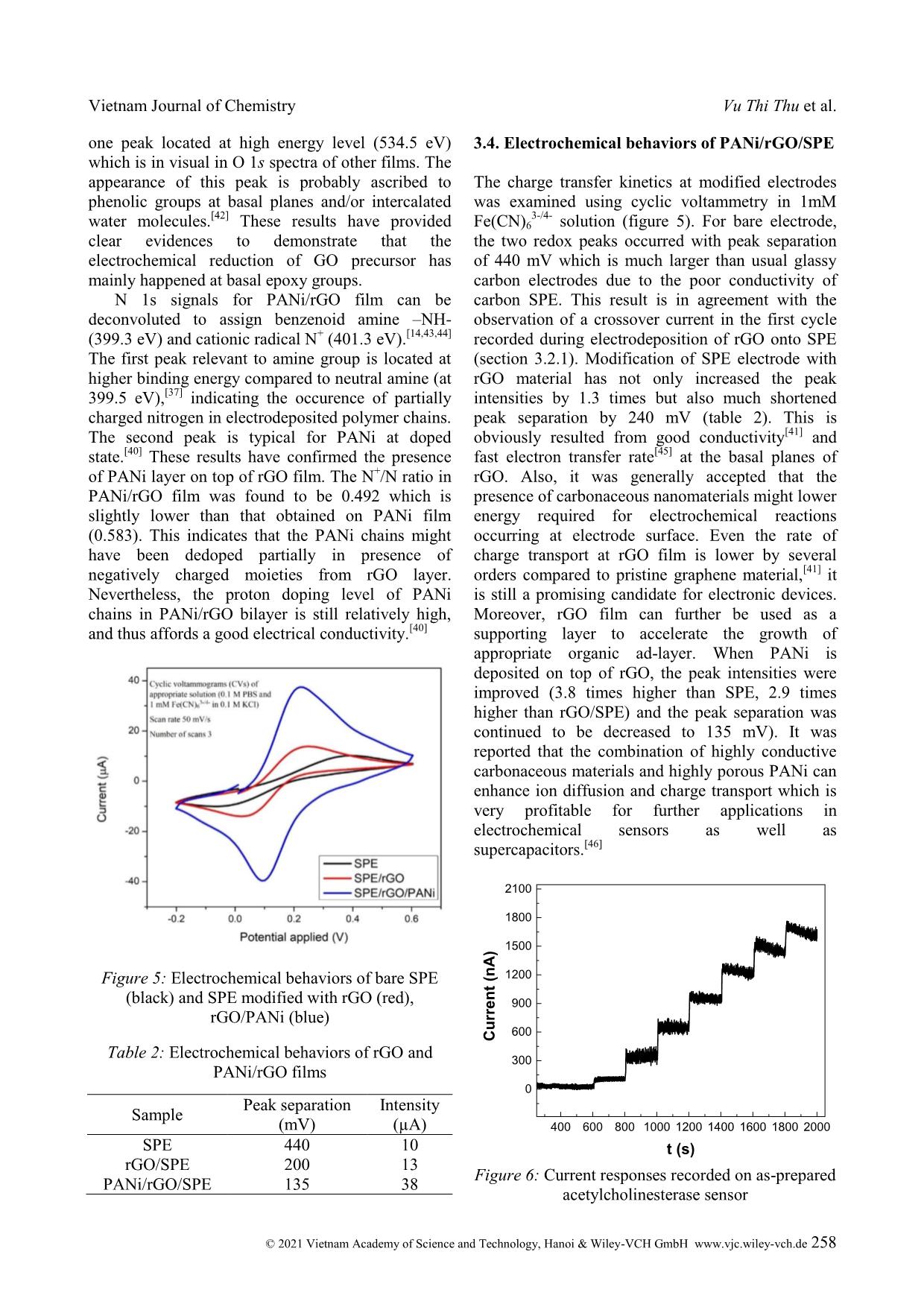
Trang 6
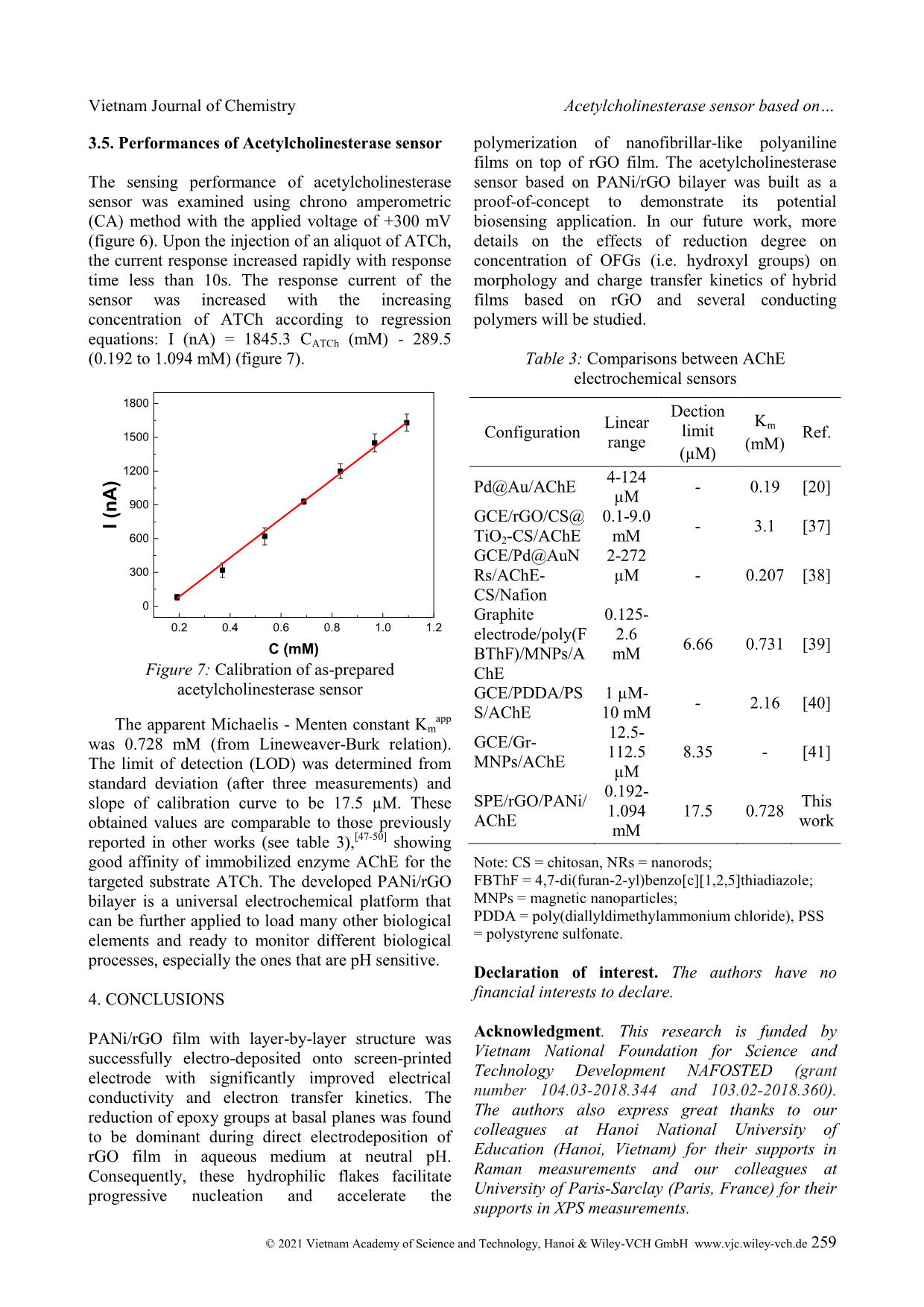
Trang 7
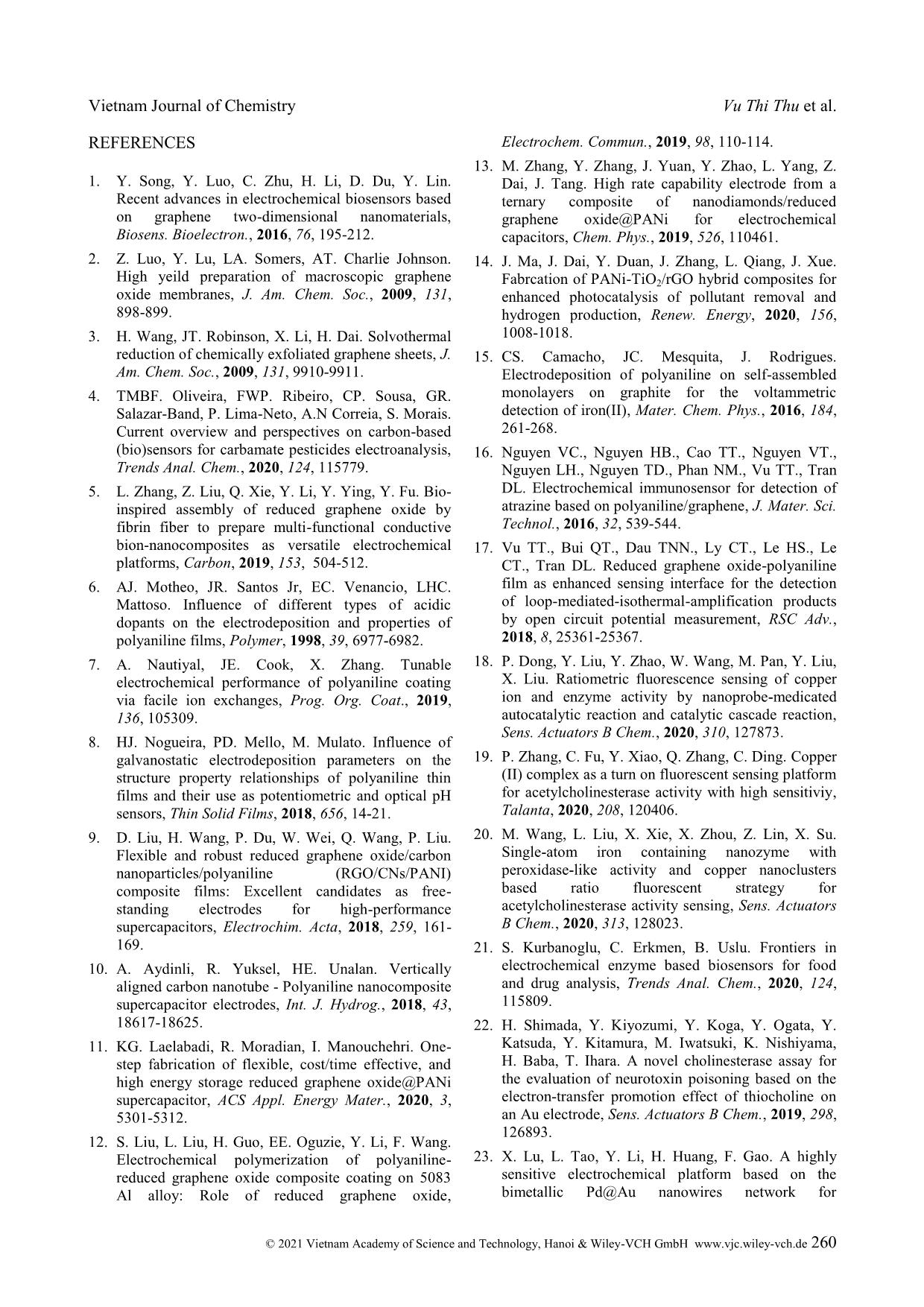
Trang 8
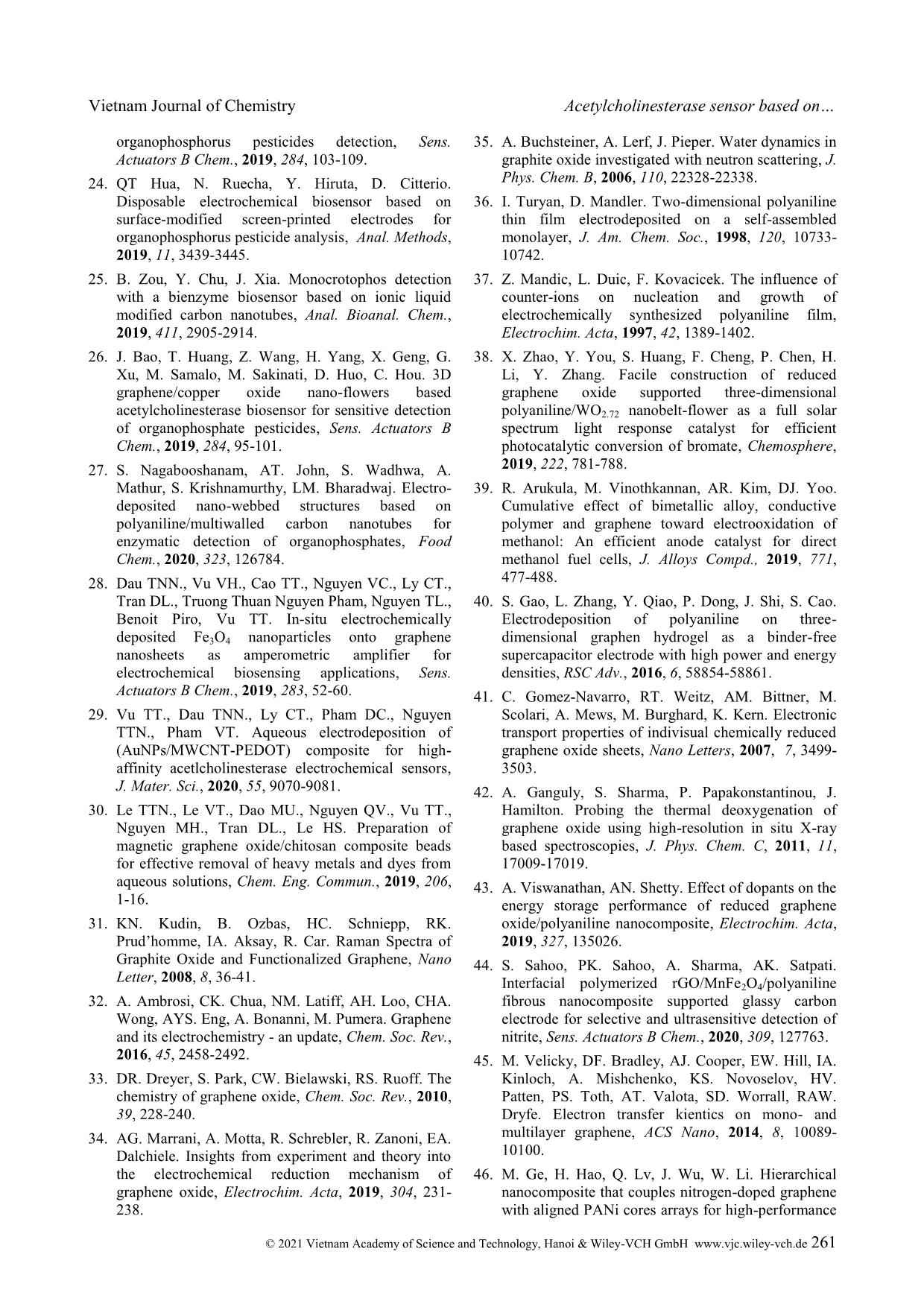
Trang 9
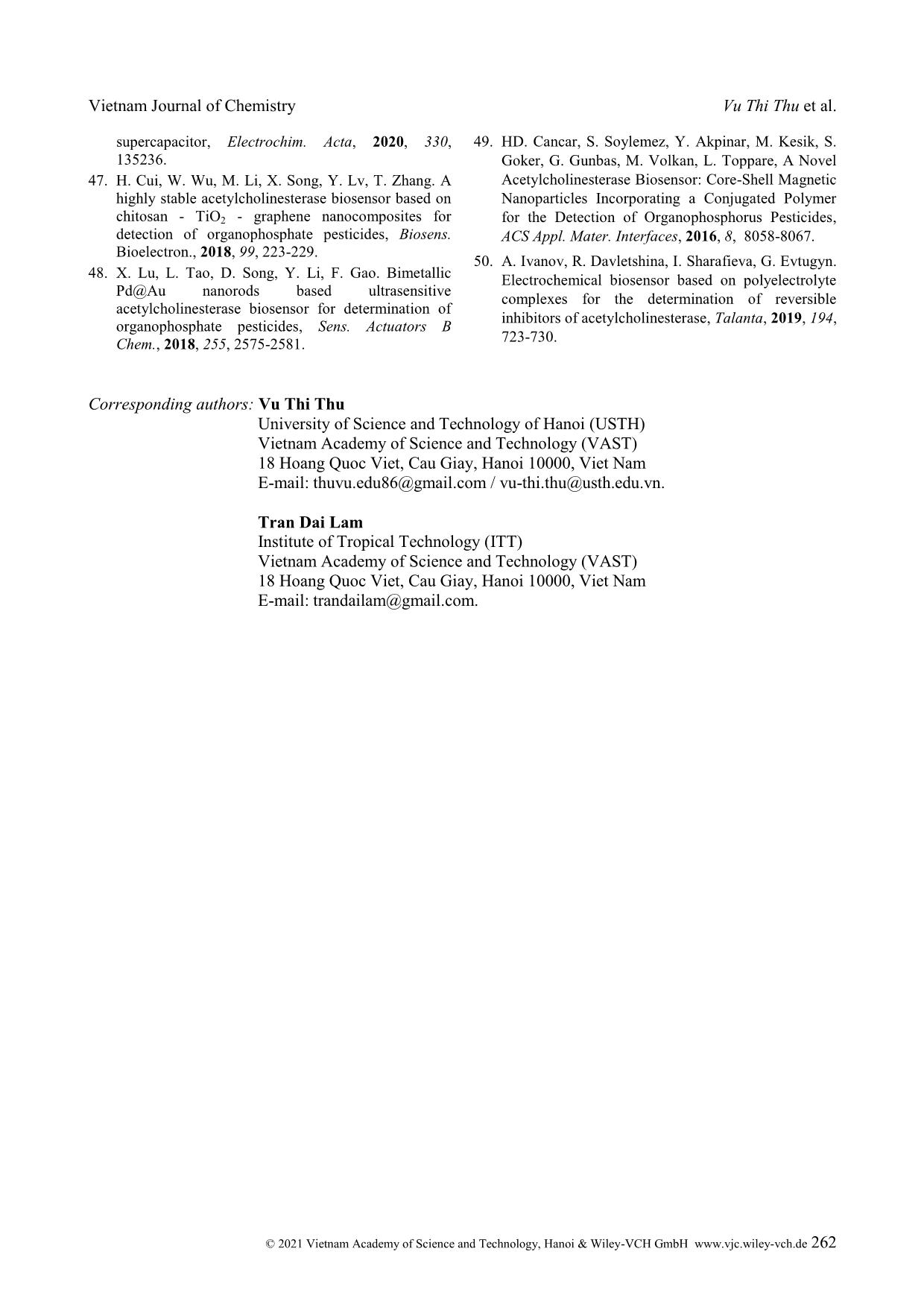
Trang 10
Tóm tắt nội dung tài liệu: Acetylcholinesterase sensor based on PANi/rGO film electrochemically grown on screen-Printed electrodes
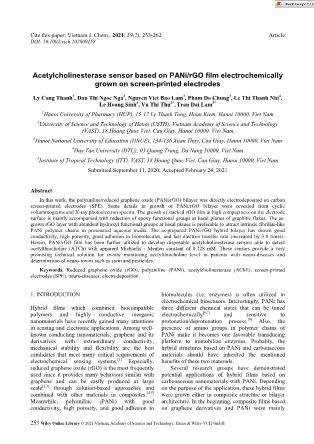
Cite this paper: Vietnam J. Chem., 2021, 59(2), 253-262 Article DOI: 10.1002/vjch.202000158 253 Wiley Online Library © 2021 Vietnam Academy of Science and Technology, Hanoi & Wiley-VCH GmbH Acetylcholinesterase sensor based on PANi/rGO film electrochemically grown on screen-printed electrodes Ly Cong Thanh 1 , Dau Thi Ngoc Nga 2 , Nguyen Viet Bao Lam 3 , Pham Do Chung 3 , Le Thi Thanh Nhi 4 , Le Hoang Sinh 4 , Vu Thi Thu 2* , Tran Dai Lam 5* 1 Hanoi University of Pharmacy (HUP), 15-17 Le Thanh Tong, Hoan Kiem, Hanoi 10000, Viet Nam 2 University of Science and Technology of Hanoi (USTH), Vietnam Academy of Science and Technology (VAST), 18 Hoang Quoc Viet, Cau Giay, Hanoi 10000, Viet Nam 3 Hanoi National University of Education (HNUE), 134-136 Xuan Thuy, Cau Giay, Hanoi 10000, Viet Nam 4 Duy Tan University (DTU), 03 Quang Trung, Da Nang 50000, Viet Nam 5 Institute of Tropical Technology (ITT), VAST, 18 Hoang Quoc Viet, Cau Giay, Hanoi 10000, Viet Nam Submitted September 11, 2020; Accepted February 24, 2021 Abstract In this work, the polyaniline/reduced graphene oxide (PANi/rGO) bilayer was directly electrodeposited on carbon screen-printed electrodes (SPE). Some details in growth of PANi/rGO bilayer were revealed from cyclic voltammograms and X-ray photoelectron spectra. The growth of stacked rGO film at high compactness on the electrode surface is mainly accompanied with reduction of epoxy functional groups at basal planes of graphitic flakes. The as- grown rGO layer with abundent hydroxyl functional groups at basal planes is preferable to attract intrinsic fibrillar-like PANi polymer chains in protonated aqueous media. The as-prepared PANi/rGO hybrid bilayer has shown good conductivity, high porosity, good adhesion to biomolecules, and fast electron transfer rate (increased by 3.8 times). Herein, PANi/rGO film has been further utilized to develop disposable acetylcholinesterase sensors able to detect acetylthiocholine (ATCh) with apparent Michaelis - Menten constant of 0.728 mM. These sensors provide a very promising technical solution for in-situ monitoring acetylthiocholine level in patients with neuro-diseases and determination of neuro-toxins such as sarin and pesticides. Keywords. Reduced graphene oxide (rGO), polyaniline (PANi), acetylcholinesterase (AChE), screen-printed electrodes (SPE), neuro-diseases, electrodeposition. 1. INTRODUCTION Hybrid films which combined biocompatible polymers and highly conductive inorganic nanomaterials have recently gained many attentions in sensing and electronic applications. Among well- known conducting nanomaterials, graphene and its derivatives with extraordinary conductivity, mechanical stability and flexibility are the best candidates that meet many critical requirements of electrochemical sensing systems. [1] Especially, reduced graphene oxide (rGO) is the most frequently used since it provides many behaviors similar with graphene and can be easily produced at large scale [2,3] through solution-based approaches and combined with other materials in composites. [4,5] Meanwhile, polyaniline (PANi) with good conductivity, high porosity, and good adhesion to biomolecules (i.e. enzymes) is often utilized in electrochemical biosensors. Interestingly, PANi has three different chemical states that can be tuned electrochemically [6,7] and sensitive to protonation/deprotonation process. [8] Also, the presence of amino groups in polymer chains of PANi make it becomes one favorable transducing platform to immobilize enzymes. Probably, the hybrid structures based on PANi and carbonaceous materials should have inherited the mentioned benefits of these two materials. Several research groups have demonstrated potential applications of hybrid films based on carbonaceous nanomaterials with PANi. Depending on the purpose of the application, these hybrid films were grown either in composite structure or bilayer architecture. In the beginning, composite films based on graphene derivatives and PANi were mainly Vietnam Journal of Chemistry Vu Thi Thu et al. © 2021 Vietnam Academy of Science and Technology, Hanoi & Wiley-VCH GmbH www.vjc.wiley-vch.de 254 utilized for developing high-performance supercapacitors in flexible energy storage devices. [9- 11] These hybrid composites also show high anti- corrosion behavior. [12] Recently, the layer-by-layer structure of hybrid films made of conducting polymers and carbonaceous materials has drawn more attentions. The assembly of the two distinct materials in two separated layers allows better control in their thickness and homogeneity. The use of graphitic material as one supporting layer provides the solution to overcome insulating nature and structural shrinkage of PANi in dedoping states. [13,14] Moreover, the addition of soft PANi material make carbonaceous materials become less rigid and more biocompatible. For instance, the PANi ad-layer electrodeposited on graphitic electrodes has been shown to improve voltammetric signals during analysis of redox probes. [15] PANi/graphene bilayer with good conductivity and fast electron transfer has been shown to be profitable in electrochemical immunosensors for tracing neuro- toxins. [16] PANi/rGO bilayer was utilized as one pH- sensitive membrane to sense protons released from gene amplification process. [17] Some suggestions on structure of PANi/rGO bilayer were previously provided but the details on growth mechanism of this hybrid bilayer is still unclear until now. Many neurodegenerative diseases (i.e, Alzheimer’s disease and Parkinson’s disease) are associated with the degeneration of the cholinergic system that is caused by abnormal AChE activity. Therefore, it is essential to develop realiable tools for monitoring the activities of AChE en ... ects of reduction degree on concentration of OFGs (i.e. hydroxyl groups) on morphology and charge transfer kinetics of hybrid films based on rGO and several conducting polymers will be studied. Table 3: Comparisons between AChE electrochemical sensors Configuration Linear range Dection limit (µM) Km (mM) Ref. Pd@Au/AChE 4-124 µM - 0.19 [20] GCE/rGO/CS@ TiO2-CS/AChE 0.1-9.0 mM - 3.1 [37] GCE/Pd@AuN Rs/AChE- CS/Nafion 2-272 µM - 0.207 [38] Graphite electrode/poly(F BThF)/MNPs/A ChE 0.125- 2.6 mM 6.66 0.731 [39] GCE/PDDA/PS S/AChE 1 µM- 10 mM - 2.16 [40] GCE/Gr- MNPs/AChE 12.5- 112.5 µM 8.35 - [41] SPE/rGO/PANi/ AChE 0.192- 1.094 mM 17.5 0.728 This work Note: CS = chitosan, NRs = nanorods; FBThF = 4,7-di(furan-2-yl)benzo[c][1,2,5]thiadiazole; MNPs = magnetic nanoparticles; PDDA = poly(diallyldimethylammonium chloride), PSS = polystyrene sulfonate. Declaration of interest. The authors have no financial interests to declare. Acknowledgment. This research is funded by Vietnam National Foundation for Science and Technology Development NAFOSTED (grant number 104.03-2018.344 and 103.02-2018.360). The authors also express great thanks to our colleagues at Hanoi National University of Education (Hanoi, Vietnam) for their supports in Raman measurements and our colleagues at University of Paris-Sarclay (Paris, France) for their supports in XPS measurements. 0.2 0.4 0.6 0.8 1.0 1.2 0 300 600 900 1200 1500 1800 I (n A ) C (mM) Vietnam Journal of Chemistry Vu Thi Thu et al. © 2021 Vietnam Academy of Science and Technology, Hanoi & Wiley-VCH GmbH www.vjc.wiley-vch.de 260 REFERENCES 1. Y. Song, Y. Luo, C. Zhu, H. Li, D. Du, Y. Lin. Recent advances in electrochemical biosensors based on graphene two-dimensional nanomaterials, Biosens. Bioelectron., 2016, 76, 195-212. 2. Z. Luo, Y. Lu, LA. Somers, AT. Charlie Johnson. High yeild preparation of macroscopic graphene oxide membranes, J. Am. Chem. Soc., 2009, 131, 898-899. 3. H. Wang, JT. Robinson, X. Li, H. Dai. Solvothermal reduction of chemically exfoliated graphene sheets, J. Am. Chem. Soc., 2009, 131, 9910-9911. 4. TMBF. Oliveira, FWP. Ribeiro, CP. Sousa, GR. Salazar-Band, P. Lima-Neto, A.N Correia, S. Morais. Current overview and perspectives on carbon-based (bio)sensors for carbamate pesticides electroanalysis, Trends Anal. Chem., 2020, 124, 115779. 5. L. Zhang, Z. Liu, Q. Xie, Y. Li, Y. Ying, Y. Fu. Bio- inspired assembly of reduced graphene oxide by fibrin fiber to prepare multi-functional conductive bion-nanocomposites as versatile electrochemical platforms, Carbon, 2019, 153, 504-512. 6. AJ. Motheo, JR. Santos Jr, EC. Venancio, LHC. Mattoso. Influence of different types of acidic dopants on the electrodeposition and properties of polyaniline films, Polymer, 1998, 39, 6977-6982. 7. A. Nautiyal, JE. Cook, X. Zhang. Tunable electrochemical performance of polyaniline coating via facile ion exchanges, Prog. Org. Coat., 2019, 136, 105309. 8. HJ. Nogueira, PD. Mello, M. Mulato. Influence of galvanostatic electrodeposition parameters on the structure property relationships of polyaniline thin films and their use as potentiometric and optical pH sensors, Thin Solid Films, 2018, 656, 14-21. 9. D. Liu, H. Wang, P. Du, W. Wei, Q. Wang, P. Liu. Flexible and robust reduced graphene oxide/carbon nanoparticles/polyaniline (RGO/CNs/PANI) composite films: Excellent candidates as free- standing electrodes for high-performance supercapacitors, Electrochim. Acta, 2018, 259, 161- 169. 10. A. Aydinli, R. Yuksel, HE. Unalan. Vertically aligned carbon nanotube - Polyaniline nanocomposite supercapacitor electrodes, Int. J. Hydrog., 2018, 43, 18617-18625. 11. KG. Laelabadi, R. Moradian, I. Manouchehri. One- step fabrication of flexible, cost/time effective, and high energy storage reduced graphene oxide@PANi supercapacitor, ACS Appl. Energy Mater., 2020, 3, 5301-5312. 12. S. Liu, L. Liu, H. Guo, EE. Oguzie, Y. Li, F. Wang. Electrochemical polymerization of polyaniline- reduced graphene oxide composite coating on 5083 Al alloy: Role of reduced graphene oxide, Electrochem. Commun., 2019, 98, 110-114. 13. M. Zhang, Y. Zhang, J. Yuan, Y. Zhao, L. Yang, Z. Dai, J. Tang. High rate capability electrode from a ternary composite of nanodiamonds/reduced graphene oxide@PANi for electrochemical capacitors, Chem. Phys., 2019, 526, 110461. 14. J. Ma, J. Dai, Y. Duan, J. Zhang, L. Qiang, J. Xue. Fabrcation of PANi-TiO2/rGO hybrid composites for enhanced photocatalysis of pollutant removal and hydrogen production, Renew. Energy, 2020, 156, 1008-1018. 15. CS. Camacho, JC. Mesquita, J. Rodrigues. Electrodeposition of polyaniline on self-assembled monolayers on graphite for the voltammetric detection of iron(II), Mater. Chem. Phys., 2016, 184, 261-268. 16. Nguyen VC., Nguyen HB., Cao TT., Nguyen VT., Nguyen LH., Nguyen TD., Phan NM., Vu TT., Tran DL. Electrochemical immunosensor for detection of atrazine based on polyaniline/graphene, J. Mater. Sci. Technol., 2016, 32, 539-544. 17. Vu TT., Bui QT., Dau TNN., Ly CT., Le HS., Le CT., Tran DL. Reduced graphene oxide-polyaniline film as enhanced sensing interface for the detection of loop-mediated-isothermal-amplification products by open circuit potential measurement, RSC Adv., 2018, 8, 25361-25367. 18. P. Dong, Y. Liu, Y. Zhao, W. Wang, M. Pan, Y. Liu, X. Liu. Ratiometric fluorescence sensing of copper ion and enzyme activity by nanoprobe-medicated autocatalytic reaction and catalytic cascade reaction, Sens. Actuators B Chem., 2020, 310, 127873. 19. P. Zhang, C. Fu, Y. Xiao, Q. Zhang, C. Ding. Copper (II) complex as a turn on fluorescent sensing platform for acetylcholinesterase activity with high sensitiviy, Talanta, 2020, 208, 120406. 20. M. Wang, L. Liu, X. Xie, X. Zhou, Z. Lin, X. Su. Single-atom iron containing nanozyme with peroxidase-like activity and copper nanoclusters based ratio fluorescent strategy for acetylcholinesterase activity sensing, Sens. Actuators B Chem., 2020, 313, 128023. 21. S. Kurbanoglu, C. Erkmen, B. Uslu. Frontiers in electrochemical enzyme based biosensors for food and drug analysis, Trends Anal. Chem., 2020, 124, 115809. 22. H. Shimada, Y. Kiyozumi, Y. Koga, Y. Ogata, Y. Katsuda, Y. Kitamura, M. Iwatsuki, K. Nishiyama, H. Baba, T. Ihara. A novel cholinesterase assay for the evaluation of neurotoxin poisoning based on the electron-transfer promotion effect of thiocholine on an Au electrode, Sens. Actuators B Chem., 2019, 298, 126893. 23. X. Lu, L. Tao, Y. Li, H. Huang, F. Gao. A highly sensitive electrochemical platform based on the bimetallic Pd@Au nanowires network for Vietnam Journal of Chemistry Acetylcholinesterase sensor based on © 2021 Vietnam Academy of Science and Technology, Hanoi & Wiley-VCH GmbH www.vjc.wiley-vch.de 261 organophosphorus pesticides detection, Sens. Actuators B Chem., 2019, 284, 103-109. 24. QT Hua, N. Ruecha, Y. Hiruta, D. Citterio. Disposable electrochemical biosensor based on surface-modified screen-printed electrodes for organophosphorus pesticide analysis, Anal. Methods, 2019, 11, 3439-3445. 25. B. Zou, Y. Chu, J. Xia. Monocrotophos detection with a bienzyme biosensor based on ionic liquid modified carbon nanotubes, Anal. Bioanal. Chem., 2019, 411, 2905-2914. 26. J. Bao, T. Huang, Z. Wang, H. Yang, X. Geng, G. Xu, M. Samalo, M. Sakinati, D. Huo, C. Hou. 3D graphene/copper oxide nano-flowers based acetylcholinesterase biosensor for sensitive detection of organophosphate pesticides, Sens. Actuators B Chem., 2019, 284, 95-101. 27. S. Nagabooshanam, AT. John, S. Wadhwa, A. Mathur, S. Krishnamurthy, LM. Bharadwaj. Electro- deposited nano-webbed structures based on polyaniline/multiwalled carbon nanotubes for enzymatic detection of organophosphates, Food Chem., 2020, 323, 126784. 28. Dau TNN., Vu VH., Cao TT., Nguyen VC., Ly CT., Tran DL., Truong Thuan Nguyen Pham, Nguyen TL., Benoit Piro, Vu TT. In-situ electrochemically deposited Fe3O4 nanoparticles onto graphene nanosheets as amperometric amplifier for electrochemical biosensing applications, Sens. Actuators B Chem., 2019, 283, 52-60. 29. Vu TT., Dau TNN., Ly CT., Pham DC., Nguyen TTN., Pham VT. Aqueous electrodeposition of (AuNPs/MWCNT-PEDOT) composite for high- affinity acetlcholinesterase electrochemical sensors, J. Mater. Sci., 2020, 55, 9070-9081. 30. Le TTN., Le VT., Dao MU., Nguyen QV., Vu TT., Nguyen MH., Tran DL., Le HS. Preparation of magnetic graphene oxide/chitosan composite beads for effective removal of heavy metals and dyes from aqueous solutions, Chem. Eng. Commun., 2019, 206, 1-16. 31. KN. Kudin, B. Ozbas, HC. Schniepp, RK. Prud’homme, IA. Aksay, R. Car. Raman Spectra of Graphite Oxide and Functionalized Graphene, Nano Letter, 2008, 8, 36-41. 32. A. Ambrosi, CK. Chua, NM. Latiff, AH. Loo, CHA. Wong, AYS. Eng, A. Bonanni, M. Pumera. Graphene and its electrochemistry - an update, Chem. Soc. Rev., 2016, 45, 2458-2492. 33. DR. Dreyer, S. Park, CW. Bielawski, RS. Ruoff. The chemistry of graphene oxide, Chem. Soc. Rev., 2010, 39, 228-240. 34. AG. Marrani, A. Motta, R. Schrebler, R. Zanoni, EA. Dalchiele. Insights from experiment and theory into the electrochemical reduction mechanism of graphene oxide, Electrochim. Acta, 2019, 304, 231- 238. 35. A. Buchsteiner, A. Lerf, J. Pieper. Water dynamics in graphite oxide investigated with neutron scattering, J. Phys. Chem. B, 2006, 110, 22328-22338. 36. I. Turyan, D. Mandler. Two-dimensional polyaniline thin film electrodeposited on a self-assembled monolayer, J. Am. Chem. Soc., 1998, 120, 10733- 10742. 37. Z. Mandic, L. Duic, F. Kovacicek. The influence of counter-ions on nucleation and growth of electrochemically synthesized polyaniline film, Electrochim. Acta, 1997, 42, 1389-1402. 38. X. Zhao, Y. You, S. Huang, F. Cheng, P. Chen, H. Li, Y. Zhang. Facile construction of reduced graphene oxide supported three-dimensional polyaniline/WO2.72 nanobelt-flower as a full solar spectrum light response catalyst for efficient photocatalytic conversion of bromate, Chemosphere, 2019, 222, 781-788. 39. R. Arukula, M. Vinothkannan, AR. Kim, DJ. Yoo. Cumulative effect of bimetallic alloy, conductive polymer and graphene toward electrooxidation of methanol: An efficient anode catalyst for direct methanol fuel cells, J. Alloys Compd., 2019, 771, 477-488. 40. S. Gao, L. Zhang, Y. Qiao, P. Dong, J. Shi, S. Cao. Electrodeposition of polyaniline on three- dimensional graphen hydrogel as a binder-free supercapacitor electrode with high power and energy densities, RSC Adv., 2016, 6, 58854-58861. 41. C. Gomez-Navarro, RT. Weitz, AM. Bittner, M. Scolari, A. Mews, M. Burghard, K. Kern. Electronic transport properties of indivisual chemically reduced graphene oxide sheets, Nano Letters, 2007, 7, 3499- 3503. 42. A. Ganguly, S. Sharma, P. Papakonstantinou, J. Hamilton. Probing the thermal deoxygenation of graphene oxide using high-resolution in situ X-ray based spectroscopies, J. Phys. Chem. C, 2011, 11, 17009-17019. 43. A. Viswanathan, AN. Shetty. Effect of dopants on the energy storage performance of reduced graphene oxide/polyaniline nanocomposite, Electrochim. Acta, 2019, 327, 135026. 44. S. Sahoo, PK. Sahoo, A. Sharma, AK. Satpati. Interfacial polymerized rGO/MnFe2O4/polyaniline fibrous nanocomposite supported glassy carbon electrode for selective and ultrasensitive detection of nitrite, Sens. Actuators B Chem., 2020, 309, 127763. 45. M. Velicky, DF. Bradley, AJ. Cooper, EW. Hill, IA. Kinloch, A. Mishchenko, KS. Novoselov, HV. Patten, PS. Toth, AT. Valota, SD. Worrall, RAW. Dryfe. Electron transfer kientics on mono- and multilayer graphene, ACS Nano, 2014, 8, 10089- 10100. 46. M. Ge, H. Hao, Q. Lv, J. Wu, W. Li. Hierarchical nanocomposite that couples nitrogen-doped graphene with aligned PANi cores arrays for high-performance Vietnam Journal of Chemistry Vu Thi Thu et al. © 2021 Vietnam Academy of Science and Technology, Hanoi & Wiley-VCH GmbH www.vjc.wiley-vch.de 262 supercapacitor, Electrochim. Acta, 2020, 330, 135236. 47. H. Cui, W. Wu, M. Li, X. Song, Y. Lv, T. Zhang. A highly stable acetylcholinesterase biosensor based on chitosan - TiO2 - graphene nanocomposites for detection of organophosphate pesticides, Biosens. Bioelectron., 2018, 99, 223-229. 48. X. Lu, L. Tao, D. Song, Y. Li, F. Gao. Bimetallic Pd@Au nanorods based ultrasensitive acetylcholinesterase biosensor for determination of organophosphate pesticides, Sens. Actuators B Chem., 2018, 255, 2575-2581. 49. HD. Cancar, S. Soylemez, Y. Akpinar, M. Kesik, S. Goker, G. Gunbas, M. Volkan, L. Toppare, A Novel Acetylcholinesterase Biosensor: Core-Shell Magnetic Nanoparticles Incorporating a Conjugated Polymer for the Detection of Organophosphorus Pesticides, ACS Appl. Mater. Interfaces, 2016, 8, 8058-8067. 50. A. Ivanov, R. Davletshina, I. Sharafieva, G. Evtugyn. Electrochemical biosensor based on polyelectrolyte complexes for the determination of reversible inhibitors of acetylcholinesterase, Talanta, 2019, 194, 723-730. Corresponding authors: Vu Thi Thu University of Science and Technology of Hanoi (USTH) Vietnam Academy of Science and Technology (VAST) 18 Hoang Quoc Viet, Cau Giay, Hanoi 10000, Viet Nam E-mail: thuvu.edu86@gmail.com / vu-thi.thu@usth.edu.vn. Tran Dai Lam Institute of Tropical Technology (ITT) Vietnam Academy of Science and Technology (VAST) 18 Hoang Quoc Viet, Cau Giay, Hanoi 10000, Viet Nam E-mail: trandailam@gmail.com.
File đính kèm:
acetylcholinesterase_sensor_based_on_panirgo_film_electroche.pdf